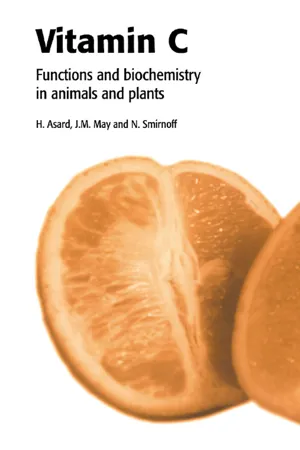
eBook - ePub
Vitamin C
Its Functions and Biochemistry in Animals and Plants
This is a test
- 344 pages
- English
- ePUB (mobile friendly)
- Available on iOS & Android
eBook - ePub
Book details
Book preview
Table of contents
Citations
About This Book
Vitamin C (ascorbic acid) is a key vitamin to animals and plants. This book looks at all aspects of vitamin C; its chemical and biochemical properties, its role in various plants and animals and its effect on our health. Written by an international team of experts, together they represent much of the expertise on vitamin C throughout the world.
Frequently asked questions
At the moment all of our mobile-responsive ePub books are available to download via the app. Most of our PDFs are also available to download and we're working on making the final remaining ones downloadable now. Learn more here.
Both plans give you full access to the library and all of Perlego’s features. The only differences are the price and subscription period: With the annual plan you’ll save around 30% compared to 12 months on the monthly plan.
We are an online textbook subscription service, where you can get access to an entire online library for less than the price of a single book per month. With over 1 million books across 1000+ topics, we’ve got you covered! Learn more here.
Look out for the read-aloud symbol on your next book to see if you can listen to it. The read-aloud tool reads text aloud for you, highlighting the text as it is being read. You can pause it, speed it up and slow it down. Learn more here.
Yes, you can access Vitamin C by Han Asard,James May,Nicholas Smirnoff in PDF and/or ePUB format, as well as other popular books in Biological Sciences & Cell Biology. We have over one million books available in our catalogue for you to explore.
Information
1
Ascorbate biosynthesis a diversity of pathways
1.1
Introduction
Introduction
1.1.1
The chemistry of ascorbate
The chemistry of ascorbate
In this chapter we review the pathways of ascorbate (ASC) biosynthesis, consider the factors controlling its rate of synthesis and accumulation and review progress in manipulating ASC synthesis. L-Ascorbate (vitamin C; L-threo-hex-2-enono-1,4-lactone) is a hexose sugar derivative (Figure 1.1). The key part of the molecule in relation to its biological activity is the ene-diol group at carbon atoms 2 and 3. This group gives ASC its acidic and reducing (antioxidant) properties, as it can both ionize (pKa=4.17 and 11.17) and readily donate electrons. The ene-diol group provides the characteristic UV absorption maximum of ASC (undissociated form 245 nm; monodissociated form 265 nm). It is soluble in water (0.33 g-ml−1), much less so in ethanol (0.033 g-ml−1) and insolublein lipophilic solvents (Jaffe, 1984). Further information on the chemistry of ASC can be found in the chapters by Buettner and Schafer (Chapter 10), and De Tullio (Chapter 9) in this volume and in Davies et al. (1991).
The oxidation of ASC is catalyzed by transition metal ions such as copper (II) or iron (III) that act as electron acceptors from ASC. ASC also reacts with a range of reactive oxygen species (ROS) such as hydrogen peroxide, superoxide and singlet oxygen, which is the basis of its antioxidant action. Reduction of hydrogen peroxide is catalyzed by ASC peroxidase (APX), an enzyme that is particularly prominent in plants (Shigeoka et al., 2002; see Foyer, Chapter 4). The role of ASC as a cofactor for a range of oxygenase and hydroxylase enzymes is also dependent on its reducing activity (see De Tullio, Chapter 9). Upon oxidation, by loss of one electron, the monodehydroascorbate (MDHA) radical is formed and this is usually the initial product of ASC oxidation in biological systems (Smirnoff, 1996; Noctor and Foyer, 1998). It is sometimes referred to as the ascorbate free radical (AFR). The key property of MHDA is that it is a relatively stable radical, which means that it can scavenge ROS and other free radicals without forming an equally reactive and destructive product (Sturgeon et al.,1998). MDHA is recycled back to ASC in two ways.
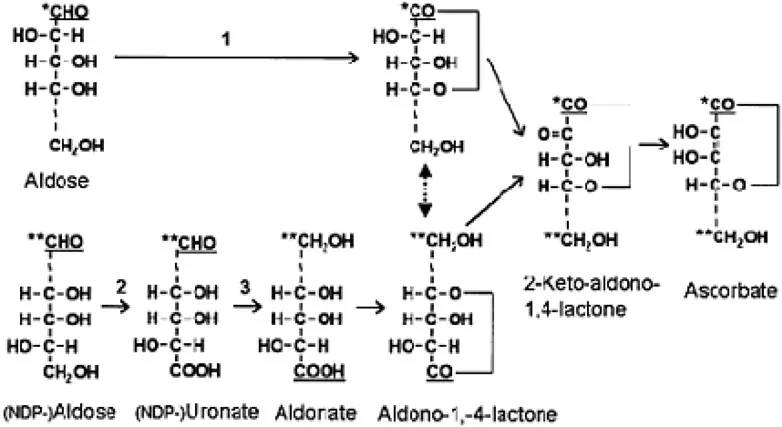
Figure 1.1: Two routes for the biosynthesis of ascorbate and its analogs via aldono-1,4lactones.
The sugars and their derivatives are represented by straight chains rather than their actual ring structures for clarity. Carbon atom 1 (C1) is underlined. In the upper pathway (direct/retention/noninversion pathway) C1, labeled by a single asterisk, is oxidized and becomes C1 of ASC (step 1). In the lower pathway (inversion pathway), sequential oxidation at C6 (step 2) and reduction at C1 (step 3) results in C1, labeled by a double asterisk, becoming C6 of the aldono-l,4-lactone. The double-headed arrow indicates that the aldono-l,4-lactones produced from each pathway are identical. In the final step the aldono-l,4-lactone is oxidized at C2 forming a 2-keto intermediate that spontaneously enolizes to ASC. The dotted lines in the carbon skeletons indicate a variable number of C atoms.
Firstly two MDHA molecules can disproportionate to form ASC and dehydroascorbate (DHA). DHA is relatively unstable, as it is subject to irreversible opening of the lactone ring to form 2,3-diketogulonic acid. DHA can be reduced back to ASC by thiols. The reaction is rapid at high pH but is also catalyzed by a glutathione-dependent DHA reductase and at a lower rate by thioredoxins and trypsin inhibitors (Morell et al., 1997), thioredoxin reductase (May et al., 1997), thiol transferase and protein disulfide isomerase (Wells et al., 1990). MDHA can also be reduced directly back to ASC by a range of mechanisms, including NAD(P)H-dependent monodehydroascorbate reductases, cytochrome b5 (Lee et al., 2001, see May and Asard, Chapter 8, and Villalba et al., Chapter 7) and by photosystem I in chloroplasts (Asada, 1999). The reactions that regenerate ASC from its oxidized forms are key to maintaining the ASC concentration of plant and animal tissues and are dealt with by May and Asard. ASC also interacts with the lipophilic antioxidant α-tocopherol (vitamin E) by regenerating it from its oxidized radical form (Asada, 1999; Smirnoff, 2000; see Buettner and Schaffer, Chapter 10).
1.1.2
Extraction and assay of ascorbate from biological samples
Extraction and assay of ascorbate from biological samples
There is a huge literature on methods for extracting and assaying ASC and DHA from biological samples and foodstuffs (Davey et al., 2000). The key issue is to avoid oxidation of ASC and degradation of DHA. This is achieved by using acidic extractants (e.g., metaphosphoric acid, perchloric acid or trichloroacetic acid) and an agent that binds transition metal ions (e.g., EDTA, oxalic acid or metaphosphoric acid). Assays for ASC include UV/colorimetric methods and chromatography. The change in UV absorbance after specifically oxidizing ASC with ASC oxidase (AO) is commonly used (Hewitt and Dickes, 1961). The ability of ASC to reduce Fe3+ in acidic solution with subsequent colorimetric determination of Fe2+, for example by the red complex formed with bipyridyl, is a convenient but less specific assay (Kampfenkel et al., 1995). In both cases ASC plus DHA is determined by first reducing DHA to ASC with a thiol reagent (e.g., dithiothreitol or homocysteine). DHA can be detected by its coloured 2,4-dinitrophenylhydrazine derivative of its de-lactonized product 2,3-diketogulonate (Roe and Kuether, 1943). Chromatography, recently mostly high pressure liquid chromatography (HPLC), can be used to increase the sensitivity and specificity of ASC determination. lon exchange, reverse phase and ion pairing separations along with UV or electrochemical detection (which increases specificity when interfering compounds are present) have been used (Kimoto et al., 1997; Kall and Andersen, 1999). DHA is determined by prior reduction to ASC or as the 2,4-di-nitrophenylhydrazine derivative of its de-lactonized product 2,3-diketogulonate. ASC can also be oxidized before chromatography and determined as DHA. A number of chromatographic methods (e.g., thin layer chromatography on cellulose and borate impregnated silica plates) can distinguish between ASC and its 5-epimer D-araboascorbate (Roomi and Tsao, 1998). Given the existence of ASC analogs and conjugates, it is advisable to check for these by chromatography when investigating an organism from a previously uninvestigated taxonomic group. MDHA, being a radical, can be detected in vivo by electron paramagnetic resonance spectroscopy (Heber et al., 1996; Hideg et al., 1997) although this approach is insensitive because oxidative stress must be applied to see the signals clearly. The development of specific fluorescent probes for ASC or DHA that could be used for in vivo localization and quantification, as can be done for glutathione, would greatly advance our understanding of ASC function.
1.1.3
The occurrence of ascorbate and ascorbate analogs
The occurrence of ascorbate and ascorbate analogs
ASC is synthesized by animals, green plants and by members of those protistan phyla that have been investigated. However in a few groups, notably guinea pigs, humans and other primates, loss of a functional form of the final enzyme of the biosynthetic pathway (L-gulonolactone oxidase) results in a dietary requirement for ASC. In contrast to the previous groups, most fungi synthesize a C5 analog, D-erythroascorbate, although some species may also synthesize 6-deoxyascorbate (Sections 1.5.2 and 1.5.3). The 5-epimer of ASC, D-araboascorbate, is found in one fungal genus, Penicillium (Section 1.5.4). The occurrence of ASC, or its analogs, in prokaryotes has not been systematically studied: it appears to be generally absent but has been reported in some cyanobacteria (Wahal et al., 1973; Aaronson et al., 1977). ASC can form conjugates at C2 with various anions (e.g., sulfate, phosphate and fatty acids; Jaffe, 1984). These are more stable than ASC because the ene-diol group is protected and they are used in food supplements. There are few reports of these in nature other than in the brine shrimp, which contains ascorbate-2-hydrogen sulphate (Tolbert et al., 1975). Its function is unknown though an ascorbate-2-sulfate sulfohydrolase has been described (Dabrowski et al., 1993). The 5-O-glycosides of the ASC analogs D-erythroascorbate and 6-deoxyascorbate occur in fungi (Section 1.5). In addition to this diversity of ASC analogs, the biosynthetic pathway of L-ASC itself also differs between Kingdoms.
1.2
Ascorbate biosynthesis in mammals
Ascorbate biosynthesis in mammals
1.2.1
Aldonolactones are the precursors of ascorbate in all known cases
Aldonolactones are the precursors of ascorbate in all known cases
Although the pathways of ASC biosynthesis differ between the major groups of organisms, the immediate precursor is always an aldono-l,4-lactone. Aldonolactones are derived from the corresponding aldose sugar, which is oxidized at Cl to produce an aldonic acid. Rearrangement of the ring structure results in the carboxylate group of the aldonic acid forming a lactone ring with C4 (Figure 1.1). ASC is formed by oxidation of the aldonolactone at C2, followed by spontaneous formation of an ene-diol group.
1.2.2
The biosynthetic pathway of ascorbate in mammals
The biosynthetic pathway of ascorbate in mammals
ASC biosynthesis is apparently localized in the liver in mammals. In egg-laying mammals, reptiles and amphibians the kidney is probably the site of synthesis, whereas in birds it may be synthesized in either or both organs (Chatterjee, 1973). The pathway is illustrated in Figure 1.2. The detailed evidence for this pathway, and the properties of the enzymes involved, are reviewed in more detail by Burns and Conney (1960) and Smirnoff (2001). The aldonolactone precursor of ASC in mammals is L-gulono-l,4-lactone (L-GuiL). It is readily converted to ASC when supplied to rats (Isherwood et al., 1954). The enzyme catalyzing this conversion, L-gulono-l,4-lactone oxidase (L-GulLO), is membrane bound and associated with the endoplasmic reticulum. ASC accumulates inside microsomal vesicles supplied with L-GulL, so the enzyme may be localized on the lumenal side (Puskas et al., 1998). It has a covalently bound FAD cofactor and paradoxically the other reaction product is hydrogen peroxide, so a high rate of ASC synthesis also generates an oxidant. L-GulLO is the only mammalian ASC biosynthesis enzyme to have been studied in any detail. Presumably the reason for this focus is that loss of a functional L-GulLO gene accounts for their inability to synthesize ASC (see Section 1.2.3).
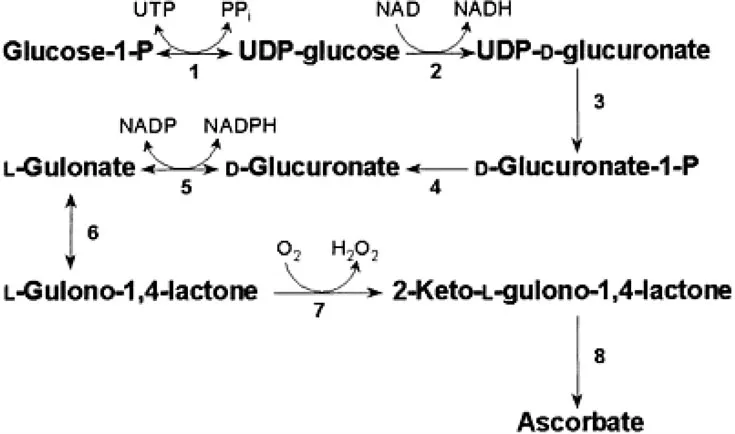
Figure 1.2: The biosynthetic pathway of ascorbate in mammals. (1) UDPglucose pyrophosphorylase. (2) UDP-glucose dehydrogenase. (3) UDP-glucuronate pyrophosphatase (speculative). (4) Glucuronate-1-P phosphatase (speculative). (5) Glucuronate reductase. (6) LGulono-l,4-lactone hydrolase (aldonolactonase, lactonase I). (7) LG...
Table of contents
- Cover
- Half Title
- Title Page
- Copyright Page
- Table of Contents
- Contributors
- Acknowledgements
- Abbrevations
- Introduction
- 1. Ascorbate biosynthesis: a diversity of pathways
- 2. Ascorbic acid catabolism: breakdown pathways in animals and plants
- 3. The biotechnology of ascorbic acid manufacture
- 4. The role of ascorbic acid in defense networks and signaling in plants
- 5. Ascorbate and plant growth: from germination to cell death
- 6. Vitamin C transport in animals and plants
- 7. Membrane redox proteins involved in ascorbate-mediated reactions
- 8. Ascorbate recycling
- 9. How does ascorbic acid prevent scurvy? A survey of the nonantioxidant functions of vitamin C
- 10. Ascorbate as an antioxidant
- 11. Vitamin C and oxidative damage to DNA
- 12. Vitamin C status declines with age
- 13. Ascorbic acid in the central nervous system: uptake, distribution and functions
- 14. Physiology of vitamin C in neutrophils during inflammation
- 15. Ascorbic acid in atherosclerosis
- 16. Dietary allowances for vitamin C: Recommended Dietary Allowances and optimal nutrient ingestion
- Index