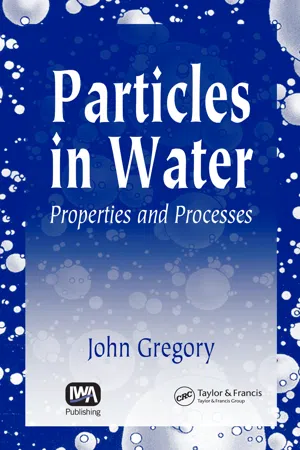
This is a test
- 200 pages
- English
- ePUB (mobile friendly)
- Available on iOS & Android
eBook - ePub
Book details
Book preview
Table of contents
Citations
About This Book
Based on the author's more than 35 years of experience, Particles in Water: Properties and Processes examines particles and their behavior in water systems. The book offers clear and accessible methods for characterizing a range of particles both individually and as aggregates. The author delineates the principles for understanding particle
Frequently asked questions
At the moment all of our mobile-responsive ePub books are available to download via the app. Most of our PDFs are also available to download and we're working on making the final remaining ones downloadable now. Learn more here.
Both plans give you full access to the library and all of Perlegoâs features. The only differences are the price and subscription period: With the annual plan youâll save around 30% compared to 12 months on the monthly plan.
We are an online textbook subscription service, where you can get access to an entire online library for less than the price of a single book per month. With over 1 million books across 1000+ topics, weâve got you covered! Learn more here.
Look out for the read-aloud symbol on your next book to see if you can listen to it. The read-aloud tool reads text aloud for you, highlighting the text as it is being read. You can pause it, speed it up and slow it down. Learn more here.
Yes, you can access Particles in Water by John Gregory in PDF and/or ePUB format, as well as other popular books in Physical Sciences & Physical & Theoretical Chemistry. We have over one million books available in our catalogue for you to explore.
Information
chapter one
Introduction
1.1 Particles in the aquatic environment
1.1.1 Origin and nature
Natural waters contain a wide range of impurities, mostly arising from weathering of rocks and soils (runoff). Contributions from human activities, especially domestic and industrial wastewaters, can also be important. Aquatic life is also a significant source of numerous constituents of natural waters.
The most fundamental point concerning impurities in the aquatic environment is the distinction between dissolved and particulate forms. Although this is a simple concept in principle, distinguishing between these forms is not always straightforward (see later in this chapter). Water is a good solvent for many substances, especially inorganic salts, and the vast majority of dissolved impurities in natural waters are of this type. The total dissolved solids (TDS) value for most fresh waters is in the range of 50â1000 mg/L (g/ m3) of which at least 90% would normally be dissolved salts. For sea water, the TDS level is of the order of 35 g/L, most of which is sodium chloride, with other salts making up nearly all of the rest.
Substances that are relatively insoluble in water may exist as small particles, which remain suspended for long periods (days or weeks). The total concentration of these impurities is known as the total suspended solids (TSS) level. In many natural waters this is very much less than the TDS level, usually of the order of 10â20 mg/L. However, there are important exceptions, especially where heavy seasonal rainf all carries large quantities of particulate material into rivers. For instance, the Yellow River in China can carry up to several g/L of suspended solids. The combined (dissolved+suspended) impurities in water are known as total solids (TS).
The main types of suspended particles found in natural waters are as follows:
- Inorganic
- Organic, including macromolecules
- Living and dead organisms
Inorganic particles result mainly from natural weathering processes and include clays, such as kaolinite and montmorillonite; oxides, including various iron oxides; and silica, calcite, and many other minerals.
Organic material mainly comes from biological degradation of plant and animal remains. Collectively, these substances are known as natural organic matter (NOM) and much of this material exists in solution. However, the size of the molecules can be quite large (macromolecules) and they have some characteristics of âparticlesâ (see later in this chapter). Common examples of âtrueâ organic particles are cell debris. NOM in water is usually measured as total organic carbon (TOC), and the soluble fraction is measured as dissolved organic carbon (DOC). The majority of the DOC falls into the class of material known as humic substances, which are responsible for the characteristic peaty-brown color of many natural waters.
Various forms of aquatic life could be considered as âparticlesâ in water, but attention is generally restricted to single-celled microorganisms. There is an enormous range of such organisms in natural waters, and, in order of increasing size, they are classified as viruses (although these are not strictly cells), bacteria, algae (including diatoms), and protozoa. Many of these may exist in water either as single cells or as much larger colonies.
Some examples of particles in natural waters are shown as micrographs in Figure 1.1. It is clear from this figure that aquatic particles have sizes over a very wide range. Particle size will be considered in the next section.
In many cases, particles are aggregated to some extent (see Figure 1.1c) so that the apparent size is larger than that of the individual particles. Aggregation of particles will be dealt with in some detail in later chapters. In the marine environment, large aggregates can form, which are known as âmarine snow.â These aggregates can be several millimeters in diameter and are made up of microorganisms such as bacteria, diatoms, fecal pellets, and many other components, bound together by polysaccharide tendrils. An example is shown in Figure 1.1d. Marine snow settles rapidly in the oceans and is thought to play a major role in transporting carbon and other nutrients to the sea floor.
1.1.2 Particle size ranges
The diagram in Figure 1.2 has a logarithmic scale of particle size over a range of 7 orders of magnitude from 1 mm down to 1 Ă
ngstrom unit (1 Ă
=10-10 m). The sizes range from atomic dimensions to those typical of sand grains. The diagram shows the wavelengths of various forms of electromagnetic radiation, together with typical size ranges of microorganisms and inorganic particles, such as clays. (Other inorganic particles such as ferric oxide cover a similar size range).
Particles larger than about 50 Îźm are usually visible as discrete objects by the unaided eye. Smaller particles have to be viewed microscopically. Down to about 1 Îźm an ordinary light microscope can be used, but smaller particles are difficult to resolve because they are comparable in size to the wavelength of visible light. For particles smaller than 1 Îźm, down to a few nm, the electron microscope provides good resolution because of the much shorter effective wavelength of electrons.
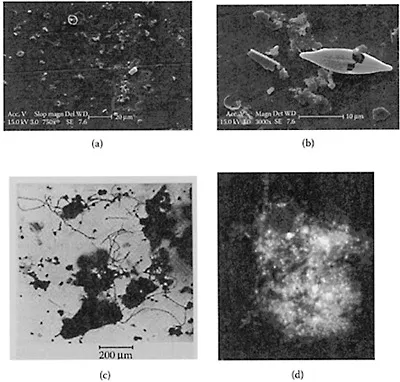
Figure 1.1 Electron micrographs of aquatic particles: (a) Sample from the river Tamar, England. (b) Same source, but higher magnification. (a and b from Doucet, F.J. et al, J. Environ. Monit., 115â121, 2005. With permission). (c) Clay and iron hydroxide particles aggregated by a network of organic filaments. (Note much larger magnification than a and b.) (By permission of Prof. J. Buffle). (d) âMarine snowâ from Monterey Bay, CA, mainly colonized by bacteria. The picture shows a 37 ÎźmÂ~27 Îźm section of a much larger aggregate. (From Azam, F. and Long, R.A., Nature, 414, 495â498, 2001. With permission).
Very small particles (around 20 nm or less) are of similar size to dissolved macromolecules, and in this region the distinction between particles and soluble matter becomes rather vague. In fact, an operational distinction between âdissolvedâ and âparticulateâ impurities is often made on the basis of a membrane filtration procedure (microfiltration; see Chapter 7). Essentially, the water sample is filtered through a membrane with a defined pore sizeâoften 0.45 Îźm. Impurities that pass through the filter (in the permeate) are conventionally regarded as âdissolved,â and those that are retained by the filter (the retentate) are defined as âparticulate.â This is an entirely arbitrary procedure, and it should be clear from Figure 1.2 that this definition would put small particles such as viruses and some clays in the âdissolvedâ category. Nevertheless, a standard procedure has some advantages, and many published listings of dissolved and particulate impurities are based on classification by membrane filtration.

Figure 1.2 Diagram showing range of particle sizes for typical aquatic particles. Also shown are wavelengths of various forms of electromagnetic radiation and appropriate particle separation processes for different size ranges. (D.A.F, dissolved air flotation.)
Another very important distinction is between colloidal and suspended (sometimes called dispersed) material. Conventionally the upper limit of the colloidal domain is set at 1 Îźm, and particles having at least 1 dimension in the size range 1â1000 nm (0.001â1 Îźm) are known as colloids. There is no sharp change of properties at a particle size of 1 Îźm, and this is an arbitrary demarcation line. However, there are some important reasons why particles are classified in this way:
- For particles smaller than about 1 Îźm, diffusion is an important transport mechanism and this tends to prevent particles from settling. Larger particles settle more rapidly and diffusion is less important, so they tend to be removed by sedimentation over time (see Chapter 2).
- At around 1 Îźm, the surface area of particles begins to become significant relative to their volume. For a sphere, the surface/volume ratio is simply 6/d, where d is the diameter. This means that, for a particle diameter of 1 Îźm and a density of 2 g/cm3, the surface area would be 3 m2/g. For a 10-nm diameter particle the surface area would be 300 m2/g. A large surface area provides more opportunity for adsorption of dissolved impurities (see later in this chapter).
- As particles become smaller, certain types of interaction between particles become more significant relative to âexternalâ forces such as gravity and fluid drag. These colloid interactions (see Chapter 4) are important for aggregation and deposition of particles in the colloidal size range.
Although there is no sudden change in properties at a certain particle size, 1 Οm represents a convenient boundary, below which these colloidal phenomena become more significant. It is also worth noting that, on a logarithmic scale, 1 Οm is about halfway between the world of macroscopic objects, such as sand grains, and the molecular domain. A famous book on colloid science by Wolfgang Ostwald, which first appeared in 1914, had the title Die Welt der vernachlässigten Dimensione (The World of Neglected Dimensions). We shall return to the subject of colloids in Section 2 of this chapter.
1.1.3 Effects of particles in water
The presence of particulate impurities in natural waters has a number of important effects, mostly detrimental, on water quality:
- Particles scatter light and give rise to turbidity in water (see Chapter 2). Inorganic particles such as clays give a noticeable cloudiness to water at concentrations from just a few mg/L. This is mainly an aesthetic problem, although there may be harmful effects of turbidity on some forms of aquatic life (e.g., predatory fish).
- Because of their relatively high surface area, as mentioned earlier, particles may adsorb certain soluble impurities from water, such as humic substances and trace metals. This can have significant effects on the transport of the adsorbed impurities because they move with the particles. One obvious effect is that dissolved substances, by adsorption on settling particles, can be transported to river, lake, or marine sediments.
- Microorganisms such as viruses and bacteria may be pathogenic (disease-causing) and so the water would be hazardous to human health. Some pathogens may attach to other particles, such as clays, and this could âshieldâ them from disinfectants used in water treatment processes.
For various reasons, particles are undesirable in drinking water, and the main aim of most common water treatment processes is particle removal (Chapter 7). The boxes in the lower part of Figure 1.2 give the ranges of particle size over which some separation techniques are appropriate.
1.2 Colloidal aspects
Subsequent chapters of this book will go into colloidal phenomena in some detail, but it is worthwhile to make some general points here.
1.2.1 Classification of colloids
The definition of colloids in terms of a size range (1â1000 nm) is convenient, but a further classification is widely used. Since the early days of colloid science, colloids in water have been divided into two distinct groupsâhydrophilic and hydrophobic (âwater lovingâ and âwater hatingâ). For nonaqueous colloids, the corresponding terms are lyophilic and lyophobic (âliquid-lovingâ and âliquid-hatingâ). We are only concerned here with aqueous colloids.
Hydrophilic colloids are essentially water-soluble macromolecules, such as proteins, gums, starch, and many synthetic polymers, generally in the size range 1â10 nm but considerably larger in the case of very-high-molecular-weight polymers. Much of the natural organic matter in water, such as humic substances, can be regarded as hydrophilic colloids. The term âcolloidâ itself, first coined by Thomas Graham (1805â1869), comes from the Greek word for âglue,â so it originally was applied to hydrophilic substances. However, Graham (known as the Father of Colloid Scienc...
Table of contents
- Cover Page
- Title Page
- Copyright Page
- Dedication
- Preface
- About the Author
- Chapter One: Introduction
- Chapter Two: Particle Size and Related Properties
- Chapter Three: Surface Charge
- Chapter Four: Colloid Interactions and Colloid Stability
- Chapter Five: Aggregation Kinetics
- Chapter Six: Coagulation and Flocculation
- Chapter Seven: Separation Methods