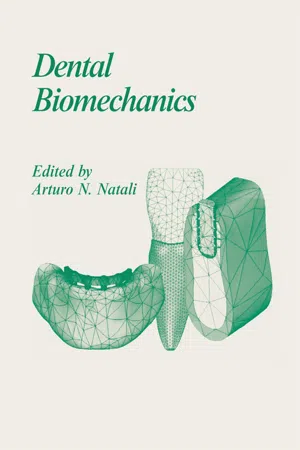
This is a test
- 304 pages
- English
- ePUB (mobile friendly)
- Available on iOS & Android
eBook - ePub
Dental Biomechanics
Book details
Book preview
Table of contents
Citations
About This Book
Dental Biomechanics provides a comprehensive, timely, and wide-reaching survey of the relevant aspects of biomechanical investigation within the dental field. Leading the reader through the mechanical analysis of dental problems in dental implants, orthodontics, and natural tooth mechanics, this book covers an increasingly important and popular sub
Frequently asked questions
At the moment all of our mobile-responsive ePub books are available to download via the app. Most of our PDFs are also available to download and we're working on making the final remaining ones downloadable now. Learn more here.
Both plans give you full access to the library and all of Perlego’s features. The only differences are the price and subscription period: With the annual plan you’ll save around 30% compared to 12 months on the monthly plan.
We are an online textbook subscription service, where you can get access to an entire online library for less than the price of a single book per month. With over 1 million books across 1000+ topics, we’ve got you covered! Learn more here.
Look out for the read-aloud symbol on your next book to see if you can listen to it. The read-aloud tool reads text aloud for you, highlighting the text as it is being read. You can pause it, speed it up and slow it down. Learn more here.
Yes, you can access Dental Biomechanics by Arturo N. Natali in PDF and/or ePUB format, as well as other popular books in Medicine & Biotechnology in Medicine. We have over one million books available in our catalogue for you to explore.
Information
1 Mechanics of bone tissue
AN Natali, RT Hart, PG Pavan, I Knets
1.1 INTRODUCTION
The present chapter deals with the mechanics of hard tissues, namely cortical bone and trabecular bone. The chapter presents various aspects of experimental activities that have been developed for the investigation of the mechanical responses of bone tissue. Tissue properties depend on the environmental conditions of the tissue, including hydration, age of specimen, etc., as well as on mechanical loading conditions, such as the rate of loading, duration of loading, etc.
It is probably superfluous to underline that experimental testing in the field of biological tissues, in particular with regard to bone tissue, requires a sophisticated approach. In fact, the limited dimensions and acquisition procedures of vitro specimens make it difficult to interpret experimental results. Moreover, the mechanical characteristics of the tissue depend on many factors, such as temperature and moisture during testing, because bone specimens are subject to the degradation caused by environmental and biochemical conditions.
Experimental testing of bone is carried out in order to get a deeper knowledge of the mechanics of bone and also to create a database to be used to perform numerical analyses of biomechanical problems (Natali and Meroi, 1989).
Numerical methods, especially the finite element method, provide powerful tools for simulating and predicting the mechanical behaviour of biological tissues. They make it possible to obtain a detailed representation of many different factors that affect the biomechanical behaviour of bone: mechanical properties, shape, loading configuration, and boundary conditions. In order to control the validity of numerical techniques, numerical results and experimental data must be compared to ensure that the models represent the real behaviour of biological structures. The results obtained validated the finite element method as a fundamental approach to investigating phenomena such as implant bone interaction or even the remodelling of bone. These in vivo responses, including bone reaction to orthodontic procedures or to dental implant practice, are aspects of bone behaviour that are particularly pertinent to dental biomechanics.
The study of bone, a unique living structural material, requires an interdisciplinary approach to understand and quantify bone functions and adaptations. Bone’s functional adaptation to mechanical loading implies the interpretation of a physiological control process. Essential components for this process include sensors for detecting mechanical response and transducers to convert these measurements to cellular response. The cellular response leads to gradual changes in bone shape and/or material properties, and once the structure has adapted, the feedback signal is diminished and further changes to shape and properties are stopped.
In order to make progress in understanding the complex response of living bone, the material and structural properties must first be quantified.
1.2 BONE
Bone is classified as a hard tissue, a definition that includes all calcified tissues. To adequately describe bone, a hierarchic scale must be identified in order to study its functional activity and properties. In fact, the macroscopic behaviour of bone is the reflection of a complex micro system relating to functions that determine the evolution of bone itself over time (Cowin, 1989). At the macroscopic level, namely for a large sample of material, bone strongly depends on the sample location and orientation as well as on specimen status and environmental factors.
At tissue level scale, bone has two distinct structures called cortical bone and trabecular bone. Cortical bone is the hard outer shell-like region of a bone and can further be classified as either primary or secondary. Primary cortical bone is made up of highly organized lamellar sheets, while secondary cortical bone is made up of sheets that are disrupted by the tunnelling of osteons centred around a Haversian canal. Trabecular bone, also called cancellous or spongy bone, is composed of calcified tissue, which forms a porous continuum.
Bone can be described as a complex of activities of three main types of cells: osteoblasts, osteoclasts and osteocytes. Osteoblasts are the cells responsible for new tissue production. Osteoclasts are related to the resorption of bone. Osteocytes are cells that are present in completely formed bone.
Mineralised microfibrils of collagen are recognised to be the components at an ultra-structural level. Their dimension is of the order of 3–5 µm. At a molecular level, three left handed helical peptide chains coiled into a triple helix form the tropocollagen molecule. These molecular structures have a dimension in the range of 1.5–280 nm (Katz, 1995) Bone tissue is continuously renewed via a complex but well coordinated sequence of activities that results first in the replacement of primary bone by secondary (osteonal) bone tissue, followed by continual renewal of the secondary bone. Bone surfaces, including not only periosteal and endocortical surfaces, but also intracortical Haversian and trabecular surfaces, are the sites for cellular activity. The bone renewal process, called remodelling, depends on a vascular supply not only for oxygen and the exchange of nutrients and minerals, but also because the pre-osteoclasts, originating in the marrow, are present in the circulation before differentiating into active osteoclasts. The multi-nucleated osteoclasts adhere to bone surfaces with a characteristic ruffled border. This allows for the creation of a (permeable) sealed microenvironment where resorption occurs, bone mineral is dissolved and the collagen and other proteins are digested (Jee, 2001).
The local coupling of bone resorption followed by new bone formation during remodelling is not yet fully understood. However, it is known that bone renewal takes place as discrete packets of cortical or trabecular bone are destroyed and replaced by a group of osteoclasts and osteoblasts referred to as a BMU (Basic Multicellular Unit). As recently described by Jee (Jee, 2001), the BMU cycle includes six consecutive stages, resting, activation, resorption, reversal, formation, mineralization, that result in the coordinated removal of bone and the construction of a new structural bone unit, either an osteon or a trabecular packet (hemiosteon). Even if the key signals for each of these steps are not fully understood, the local mechanical environment and the local chemical environment (including hormones and growth factors) are both known to be important. In addition, since the renewal process is not perfect, only about 95 per cent of the removed bone is replaced (Jee, 2001), the bone structure becomes increasingly compromised over time.
Bone can also be stimulated to change its shape and size, a process called either modelling or net remodelling. Most modelling occurs during growth with changes in bone shape and size. However, even after maturity, bone may be stimulated by altering mechanical loading or by different agents that affects change of shape and/or material properties.
1.3 EXPERIMENTAL TESTING AND RESULTS
In spite of the fact that an official codification of experimental testing procedures has not been fully defined, the preparation of specimens follows an almost standard procedure. The main problem is to obtain in vitro specimens that should have, as far as possible, the same characteristics of the tissue in vivo, especially with regard to bone hydration (Ashman, 1989). Freezing specimens is probably the most common process used to maintain the original water content in bone. Furthermore, freezing has a marginal influence on bone mechanical properties.
The method of testing traditional engineering materials is also adopted for bone, paying attention to the small dimension of the specimens taken from in vivo bones (Reilly et al., 1974). Samples of the cortical portion of bone are usually about 5/5/15 mm, with square or circular cross section. The samples of cancellous bone have similar dimensions but are usually potted in acrylic at the ends.
Several tissue characteristics are highlighted in the following description of experimental testing results for cortical and trabecular bone.
1.3.1 Anisotropic characteristics of bone tissue
The anisotropic stiffness properties of cortical bone are revealed by simply loading the specimens in different directions. Values of the elastic parameters are found, depending on the loading direction, as reported in Table 1.1. In addition, the strength of bone depends on the loading direction and differs depending on compression or tension loads. Table 1.2 shows values which are usually assumed for yield and ultimate stress. They are obtained by applying axial loading and torsional loading to cortical bone specimens taken from human femur (Cowin et al., 1989). The maximum value of the yield stress is found for compression along the main axis of a long bone and is about 180 MPa. Tensile yield stress along the same material axis is approximately 120 MPa. Yielding under torsional loading in the plane normal to the main axis of bone is close to 55 MPa.
Table 1.1 Average elastic constants for mandibular corpus in different zones
Table 1.2 Yield and ultimate stress values for human cortical bone
It is also important to outline the influence of combined loading configurations, which better characterize the in vivo conditions, on bone response. The anisotropic characteristics of trabecular bone are mostly related to the architecture of the trabecular network
1.3.2 Time dependent response
Bone shows a time dependent behaviour described as viscoelastic in certain conditions. Typical viscoelastic behaviour, such as ...
Table of contents
- COVER PAGE
- TITLE PAGE
- COPYRIGHT PAGE
- PREFACE
- CONTRIBUTORS
- 1: MECHANICS OF BONE TISSUE
- 2: MECHANICS OF PERIODONTAL LIGAMENT
- 3: COMPUTER TOMOGRAPHY FOR VIRTUAL MODELS IN DENTAL IMAGING
- 4: COMPUTER-AIDED, PRE-SURGICAL ANALYSIS FOR ORAL REHABILITATION
- 5: MATERIALS IN DENTAL IMPLANTOLOGY
- 6: DENTAL DEVICES IN TITANIUM-BASED MATERIALS VIA CASTING ROUTE
- 7: TESTING THE RELIABILITY OF DENTAL IMPLANT DEVICES
- 8: ON THE MECHANICS OF SUPERELASTIC ORTHODONTIC APPLIANCES
- 9: CLINICAL PROCEDURES FOR DENTAL IMPLANTS
- 10: CLINICAL PROCEDURES IN ORTHODONTICS
- 11: NUMERICAL APPROACH TO DENTAL BIOMECHANICS
- 12: MECHANICS OF MATERIALS