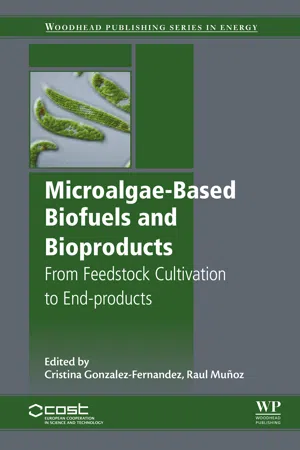
Microalgae-Based Biofuels and Bioproducts
From Feedstock Cultivation to End-Products
- 560 pages
- English
- ePUB (mobile friendly)
- Available on iOS & Android
Microalgae-Based Biofuels and Bioproducts
From Feedstock Cultivation to End-Products
About This Book
Microalgae-Based Biofuels and Bioproducts: From Feedstock Cultivation to End Products compiles contributions from authors from different areas and backgrounds who explore the cultivation and utilization of microalgae biomass for sustainable fuels and chemicals.
With a strong focus in emerging industrial and large scale applications, the book summarizes the new achievements in recent years in this field by critically evaluating developments in the field of algal biotechnology, whilst taking into account sustainability issues and techno-economic parameters. It includes information on microalgae cultivation, harvesting, and conversion processes for the production of liquid and gaseous biofuels, such as biogas, bioethanol, biodiesel and biohydrogen. Microalgae biorefinery and biotechnology applications, including for pharmaceuticals, its use as food and feed, and value added bioproducts are also covered.
This book's comprehensive scope makes it an ideal reference for both early stage and consolidated researchers, engineers and graduate students in the algal field, especially in energy, chemical and environmental engineering, biotechnology, biology and agriculture.
- Presents the most current information on the uses and untapped potential of microalgae in the production of bio-based fuels and chemicals
- Critically reviews the state-of-the-art feedstock cultivation of biofuels and bioproducts mass production from microalgae, including intermediate stages, such as harvesting and extraction of specific compounds
- Includes topics in economics and sustainability of large-scale microalgae cultivation and conversion technologies
Frequently asked questions
Information
Photobioreactors for the production of microalgae
† National Institute of Industrial Engineering and Technology, Lisbon, Portugal
‡ CNR-Institute for Ecosystem Study, Sesto Fiorentino, Italy
§ University of Antofagasta, Antofagasta, Chile
⁎⁎ Czech Academy of Sciences, Třeboň, Czech Republic
†† University of South Bohemia, České Budějovice, Czech Republic
Abstract
Keywords
1.1 Introduction
1.2 Requirements of photosynthetic microorganisms
1.2.1 Light availability
Table of contents
- Cover image
- Title page
- Table of Contents
- Copyright
- List of contributors
- Acknowledgments
- 1: Photobioreactors for the production of microalgae
- 2: Heterotrophic and mixotrophic microalgae cultivation
- 3: Microalgae cultivation in wastewater
- 4: Applications of genome-scale metabolic models of microalgae and cyanobacteria in biotechnology
- 5: Harvesting of microalgae: Overview of process options and their strengths and drawbacks
- 6: Cell disruption technologies
- 7: Biogas production from microalgae
- 8: Breakthroughs in bioalcohol production from microalgae: Solving the hurdles
- 9: Biohydrogen production from microalgae
- 10: Biodiesel from microalgae
- 11: Pyrolysis of microalgae for fuel production
- 12: Biogas upgrading using algal-bacterial processes
- 13: Synthetic biology of cyanobacteria for production of biofuels and high-value products
- 14: Biorefinery of algae: Technical and economic considerations
- 15: Microalgal proteins for feed, food and health
- 16: Microalgal fatty acids—From harvesting until extraction
- 17: Cyanobacterial toxins as a high value-added product
- 18: Trends in red biotechnology: Microalgae for pharmaceutical applications
- 19: Extraction of value-added compounds from microalgae
- 20: Economics of microalgae production
- 21: Environmental impacts of full-scale algae cultivation
- Index