
- 260 pages
- English
- ePUB (mobile friendly)
- Available on iOS & Android
Ultracold Bosonic and Fermionic Gases
About This Book
The rapidly developing topic of ultracold atoms has many actual and potential applications for condensed-matter science, and the contributions to this book emphasize these connections.
Ultracold Bose and Fermi quantum gases are introduced at a level appropriate for first-year graduate students and non-specialists such as more mature general physicists. The reader will find answers to questions like: how are experiments conducted and how are the results interpreted? What are the advantages and limitations of ultracold atoms in studying many-body physics? How do experiments on ultracold atoms facilitate novel scientific opportunities relevant to the condensed-matted community?
This volume seeks to be comprehensible rather than comprehensive; it aims at the level of a colloquium, accessible to outside readers, containing only minimal equations and limited references. In large part, it relies on many beautiful experiments from the past fifteen years and their very fruitful interplay with basic theoretical ideas. In this particular context, phenomena most relevant to condensed-matter science have been emphasized.
- Introduces ultracold Bose and Fermi quantum gases at a level appropriate for non-specialists
- Discusses landmark experiments and their fruitful interplay with basic theoretical ideas
- Comprehensible rather than comprehensive, containing only minimal equations
Frequently asked questions
Information
1 Introduction: Why so Cold?







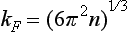

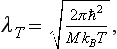

Table of contents
- Cover image
- Table of Content
- Edited by
- Copyright page
- List of Contributors
- Series Preface
- Volume Preface
- Chapter 1 Experimental Methods of Ultracold Atomic Physics
- Chapter 2 Bose Gas: Theory and Experiment
- Chapter 3 The Fermi Gases and Superfluids: Experiment and Theory
- Chapter 4 Low-Dimensional Atomic Bose Gases
- Chapter 5 Ultracold Atoms and Molecules in Optical Lattices
- Chapter 6 Unitary Fermi Gases
- Chapter 7 Potential Insights into Nonequilibrium Behavior from Atomic Physics
- Index