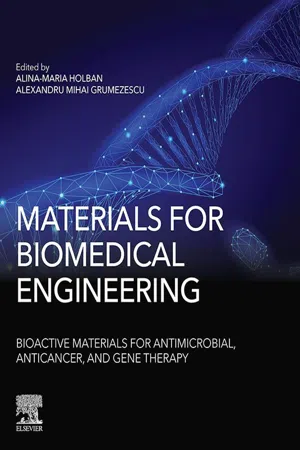
eBook - ePub
Materials for Biomedical Engineering: Bioactive Materials for Antimicrobial, Anticancer, and Gene Therapy
This is a test
- 466 pages
- English
- ePUB (mobile friendly)
- Available on iOS & Android
eBook - ePub
Materials for Biomedical Engineering: Bioactive Materials for Antimicrobial, Anticancer, and Gene Therapy
Book details
Book preview
Table of contents
Citations
About This Book
Materials for Biomedical Engineering: Bioactive Materials for Antimicrobial, Anticancer, and Gene Therapy offers an up-to-date perspective on recent research findings regarding the application and use of these materials for disease treatment and prevention. Various types of currently investigated bioactive materials, including therapeutic nanostructures and antimicrobial hydrogels are discussed, as are their properties, impact and future role in therapeutic applications. The book will be extremely useful for new researchers who want to explore more information on the use of bioactive materials or for more experienced researchers who are interested in new trends and specific applications.
- Provides knowledge on the range of bioactive materials available, enabling the reader to make optimal materials selection decisions
- Contains detailed information on current and proposed applications of the latest bioactive materials to empower readers to design innovative products and processes
- Presents a strong emphasis on chemistry and the physico-chemical characterization of these materials and their application in antimicrobial, anticancer and gene therapy
Frequently asked questions
At the moment all of our mobile-responsive ePub books are available to download via the app. Most of our PDFs are also available to download and we're working on making the final remaining ones downloadable now. Learn more here.
Both plans give you full access to the library and all of Perlego’s features. The only differences are the price and subscription period: With the annual plan you’ll save around 30% compared to 12 months on the monthly plan.
We are an online textbook subscription service, where you can get access to an entire online library for less than the price of a single book per month. With over 1 million books across 1000+ topics, we’ve got you covered! Learn more here.
Look out for the read-aloud symbol on your next book to see if you can listen to it. The read-aloud tool reads text aloud for you, highlighting the text as it is being read. You can pause it, speed it up and slow it down. Learn more here.
Yes, you can access Materials for Biomedical Engineering: Bioactive Materials for Antimicrobial, Anticancer, and Gene Therapy by Alexandru Grumezescu,Alina Maria Holban in PDF and/or ePUB format, as well as other popular books in Technologie et ingénierie & Science des matériaux. We have over one million books available in our catalogue for you to explore.
Information
Subtopic
Science des matériauxChapter 1
Therapeutic nanostructures: novel approaches
Irina Gheorghe1, 2, Carmen Curutiu1, 2 and Lia-Mara Ditu1, 2, 1Faculty of Biology, Microbiology Department, University of Bucharest, Bucharest, Romania, 2Research Institute of the University of Bucharest, Bucharest, Romania
Abstract
Nanotechnology provides new methods to improve the biological and medical processes that take place on a nanometer scale in order to achieve benefits for biology and medicine. At nanoscale dimensions, controlling the structure squarely means controlling the variation of the surface layers for higher aqueous solubility and controlling biocompatibility and bioconjugation. Currently, research efforts are underway to integrate nanoparticles in the research fields of biology and medicine. This large field offers viable support for biomedical applications for the monitoring, diagnosis, repair, and treatment of human biological systems. This chapter is an introduction to the great research field of therapeutic nanostructures, focusing on antimicrobial, antitumoral, and gene therapies.
Keywords
nanoparticles; nanotechnology; antibiotics; cancer therapy; nanocarriers; gene therapy
1.1 Nanomaterials: a viable approach to combatting multidrug-resistant bacteria
Antimicrobial resistance (AR) is an ever-growing problem in intensive care units. The number of Gram-negative bacteria resistant to one or more antibiotics is on the rise. For example, between 1986 and 2003, the ratio of Enterobacteriaceae strains resistant to ceftazidime, cefpodoxime, and cefotaxime has increased 10 times; resistant Gram-negative fermenting rods, especially Klebsiella pneumoniae and Escherichia coli, have increased by twofold (Gaynes and Edwards, 2005). Recently, a nonfermenting, Gram-negative Acinetobacter baumannii has been associated with hospital- and community-acquired infections. Similarly, other less encountered Gram-negative bacteria such as Stenotrophomonas maltophilia and Aeromonas species have been increasingly reported in abundant episodes of bacteremia, meningitis, surgical site infections, wound problems in intensive care settings, and pneumonia (Datta and Wattal, 2010).
Antibiotics are influencing the microbiota of the digestive tract, which can cause multidrug-resistant (MDR) isolates. Therefore new ways of creating biocide materials represent a very attractive field. A biocidal material is defined as a chemical substance or microorganism expected to destroy or reduce the harmful effects of organisms that can cause damage. For this reason, it is important to use an agent that does not cause resistance and has a good bactericidal property.
1.1.1 Antimicrobial resistance mechanisms in Gram-negative rods
Beta-lactams are a group of antibiotics (Table 1.1) that share a common structural feature, a highly reactive four-member, or β-lactam, ring (Donowitz and Mandell, 1988). Their antibacterial effect is achieved by interfering with the final stage of bacterial cell wall synthesis. Beta-lactam antibiotics (Table 1.1) are competitive inhibitors of the bacterial transpeptidase because of their constructional similarity to the terminal D-Ala-D-Ala motif of N-acetyl muramic acid (NAM). When a transpeptidase uses a β-lactam as a substrate, it becomes acylated and so is unavailable to perform its usual biological function and cell wall synthesis is hindered. And a result, the cell wall becomes compromised, allowing a flow of water into the cell, which then leads to cell lysis and cell death (Goffin and Ghuysen, 1998).
Table 1.1
Penicillins | Cephalosporins | Carbapenems | Monobactams | Cephamycins |
---|---|---|---|---|
Natural penicillins Benzylpenicillin (Penicillin G) Phenoxymethylpenicillin (Penicillin V) | First generation (narrow spectrum) Cephalothin Cephalexin | Meropenem Ertapenem Imipenem | Aztreonam | Cefmetazole Cefoxitin |
Extended-spectrum penicillins Aminopenicillins (Ampicillin, Amoxicillin) Carboxypenicillins (Carbenicillin, Ticarcillin) | Second generation (extended spectrum) Cefuroxime Cefamandole Cefaclor | |||
Antistaphylococcal (to target staphylococcal penicillinase producers) Cloxacillin Methicillin Oxacillin | Third generation (broad spectrum) Cefotaxime Ceftriaxone | |||
Fourth generation (broad spectrum) Cefepime Cefpirome | ||||
Fifth generation (anti-MRSA) Ceftobiprole |
Resistance to β-lactams in Enterobacteriaceae is associated with β-lactamase production, which hydrolyzes the beta-lactam ring. The Ambler classes depend on the amino acid homology—they are classified in four molecular classes, namely, A, B, C, and D. The classes A, C, and D include the serine β-lactamases, whereas class B includes metallo-beta-lactamases (MBLs). The Bush-Jacoby-Medeiros classification grouped the β-lactamases into 3 major groups and 16 subgroups (Ambler, 1980).
1.1.1.1 Extended-spectrum beta-lactamases
Extended-spectrum beta-lactamases (ESBLs) confer resistance to the penicillins, early and extended-spectrum cephalosporins, and aztreonam and are inhibited by β-lactamase inhibitors like sulbactam, clavulanic acid, and tazobactam (Bush and Fisher, 2011).
CTX-M β-lactamases were first described in Kluyvera sp. chromosomally located opposite to E. coli and Klebsiella sp., in which this gene is plasmid located. These enzymes are able to hydrolyze cefotaxime, aztreonam, and extended-spectrum cephalosporins. This group includes five subgroups, CTX-M-1, -2, -8, -9, and -25, and CTX-M-15 represents the most encountered type belonging to group 1 (Bonnet, 2004).
OXA β-lactamases (Bush-Jacoby-Medeiros group 2d and Ambler class D) were named after their oxacillin-hydrolyzing abilities; actually, they inactivate benzylpenicillin, cloxacillin, and oxacillin (Bush and Fisher, 2011). They predominantly happen in Pseudamonas aeruginosa (Weldhagen et al., 2003) but have been reported also in Enterobacteriaceae strains. OXA-1 and OXA-10 β-lactamases have only a tight hydrolytic spectrum; however; other OXA beta-lactamases are ESBLs, including OXA-11, -14, -15,-16, -28, -31, -35, and -45, as they confer resistance to cefotaxime, ceftazidime, and aztreonam. Altogether 311 OXA-type β-lactamases were discovered, including narrow-spectrum and extended-spectrum-β-lactamases (Toleman et al., 2003).
Pseudomonas ESBLs hydrolyze penicillins and cephalosporins and are inhibited by clavulanic acid. PSE-1 was first described in P. aeruginosa, as well as in Salmonella and Acinetobacter isolates (Neuhauser et al., 2003; Vahaboglu et al., 2001; Luzzaro et al., 2001; Szabó et al., 2008). VEB (Vietnam extended-spectrum β-lactamase) has 38% homology with Pseudomonas extended resistance. The gene encoding VEB-1 was found to be plasmid located and carries other resistance determinants as well (Poirel et al., 1999).
1.1.1.2 Carbapenemases
Carbapenemases are β-lactamases with a broad hydrolytic spectrum. Almost all hydrolyzable β-lactams, including the carbapenems, inactivate by the action of these enzymes (Queenan and Bush, 2007). In class A, the prevalent carbapenemase is KPC, which was mainly detected on plasmids of K. pneumoniae (Yigit et al., 2001; Nordmann et al., 2011). Previously, sporadic cases of K. pneumoniae carbapenemase producing E. coli, Enterobacter cloacae, Serratia marcescens, and Citrobacter freundii isolates were detected (Bush, 2010). K. pneumoniae carbapenemase enzymes have 15 different amino acid variants and possess hydrolytic activity on the extended-spectrum cephalosporins, carbapenems, and aztreonam (Bush and Fisher, 2011). The nonmetallo-carbapenemase (NMC), imipenem hydrolyzing β-lactamase (IMI), and S. marcescens enzyme (SME) carbapenemases belong to Ambler class A and to 2f in the Bush-Jacoby-Medeiros classification (Naas et al., 1994). All three enzymes have a broad spectrum, which includes the penicillins, early cephalosporins, carbapenems, and aztreonam (Queenan and Bush, 2007).
Ambler class B and Bush-Jacoby-Medeiros group 3a include the MBLs, capable of hydrolyzing β-lactams, including carbapenems, depending on the interaction of Zn2+ in the enzyme’s active site, and this is the side inhibited by ethylenediaminetetraacetic acid (EDTA) (Bush and Fisher, 2011; Queenan...
Table of contents
- Cover image
- Title page
- Table of Contents
- Copyright
- List of contributors
- Series preface
- Preface
- Chapter 1. Therapeutic nanostructures: novel approaches
- Chapter 2. Biodegradable antimicrobial hydrogels and their use in biomedical purposes
- Chapter 3. Antimicrobial fibers obtained by electrospinning
- Chapter 4. Hybrid films based on nonisocyanate polyurethanes with antimicrobial activity
- Chapter 5. Antimicrobial activity of green synthesized plasmonic nanoparticles
- Chapter 6. Metallic nanoparticles as a potential antimicrobial for catheters and prostheses
- Chapter 7. Core–shell nanomaterials for infection and cancer therapy
- Chapter 8. On-demand tailored mesoporous silica nanoparticles as a theranostic platform for tumor therapy
- Chapter 9. Applications of Fe3O4 magnetic-fluorescent nanoparticles in modern biomedical engineering
- Chapter 10. Gene therapy: recent progress and perspectives
- Chapter 11. Development of plasmid DNA nanoparticles for mitochondrial gene therapy
- Chapter 12. Mechanical performance of lithium disilicate: update on the latest experimental results
- Chapter 13. Polysaccharide-based hydrogels for targeted drug delivery
- Chapter 14. Metallic nanoparticles as a strategy for the treatment of infectious diseases
- Chapter 15. Nanomaterials for medical applications and their antimicrobial advantages
- Index