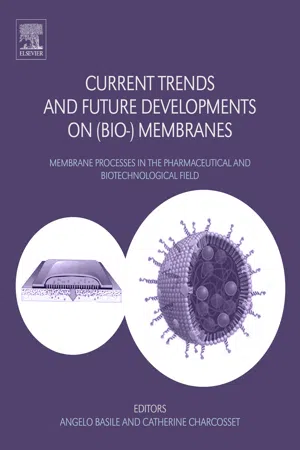
Current Trends and Future Developments on (Bio-) Membranes
Membrane Processes in the Pharmaceutical and Biotechnological Field
- 365 pages
- English
- ePUB (mobile friendly)
- Available on iOS & Android
Current Trends and Future Developments on (Bio-) Membranes
Membrane Processes in the Pharmaceutical and Biotechnological Field
About This Book
Current Trends and Future Developments on (Bio-) Membranes: Membrane Processes in the Pharmaceutical and Biotechnological field presents the main membrane techniques along with their basic principles, mode of operations, and applications. It covers well-known techniques such as ultrafiltration and membrane chromatography, while also exploring emerging membrane technologies which are finding their way in pharmaceutical and biotechnology industries, including membrane emulsification, membrane bioreactors, and solvent-resistant nanofiltration. State-of-the-art applications of membrane systems in areas such as drug delivery and virus removal are also investigated by leading experts in the field.
Current Trends and Future Developments on (Bio-) Membranes: Membrane Processes in the Pharmaceutical and Biotechnological field is a definitive reference for academics, post-graduates, and researchers in the subjects of biochemical engineering, pharmaceutics, and biotechnology. It is also useful to R&D companies and institutions in these areas, specifically those interested in bioseparations, biopurification, bioproduction, and drug delivery.
- Offers an overview of classical membrane-based separation techniques such as ultrafiltration, microfiltration and virus filtration
- Discusses emerging membrane-based separation techniques such as nofiltration in the presence of solvent, membrane emulsification and membrane crystallization
- Outlines their applications to bioseparation, biopurification and bioproduction
- Includes examples in the production of vaccines, antibiotics, biomolecules, drugs, DNA and cells
- Lists membranes systems for drug delivery like liposomes, nanocapsules and bilayer membranes
Frequently asked questions
Information
Ultra- and Microfiltration in Dairy Technology
Abstract
Keywords
g/L
g/L
g/L
g/L
Pa
Pa
Pas
°C
L/(m2h)
m
Pa
Pa
Pa
Pa
Pa
mâ 1
mâ 1
Pa
1 Introduction

Table of contents
- Cover image
- Title page
- Table of Contents
- Copyright
- Contributors
- Preface
- Chapter 1: Ultra- and Microfiltration in Dairy Technology
- Chapter 2: Microfiltration in Pharmaceutics and Biotechnology
- Chapter 3: Virus Removal and Virus Purification
- Chapter 4: Nanofiltration in the Pharmaceutical and Biopharmaceutical Technology
- Chapter 5: Purification of New Biologicals Using Membrane-Based Processes
- Chapter 6: Membrane Chromatography for Biomolecule Purification
- Chapter 7: Membrane Emulsification in Pharmaceutics and Biotechnology
- Chapter 8: Applications of Membrane Bioreactors in Biotechnology Processes
- Chapter 9: Supported Liquid Membranes in Pharmaceutics and Biotechnology
- Chapter 10: Drug Delivery With Membranes Systems
- Chapter 11: Lipid Membrane Models for Biomembrane Propertiesâ Investigation
- Index