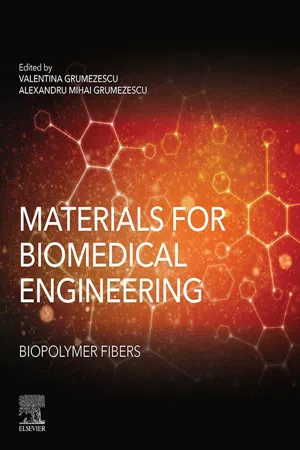
eBook - ePub
Materials for Biomedical Engineering: Biopolymer Fibers
This is a test
- 478 pages
- English
- ePUB (mobile friendly)
- Available on iOS & Android
eBook - ePub
Materials for Biomedical Engineering: Biopolymer Fibers
Book details
Book preview
Table of contents
Citations
About This Book
Materials for Biomedical Engineering: Biopolymer Fibers discusses the use of biopolymer fibers in the development of biomedical applications. It provides a recent review of the main types of polymeric fibers and their impact in biomedicine and related fields. The development of different instruments, such as sensors, medical fibers, and textiles are discussed, along with how they greatly benefited by progress made in polymeric fibers. The book provides a comprehensive and updated reference on the latest research in the field of biopolymers and their composites in relation to medical applications.
- Provides a valuable resource of recent scientific progress, highlighting the application and use of polymeric fibers in biomedical engineering that can be used by researchers, engineers and academics
- Includes novel opportunities and ideas for developing or improving technologies in biopolymers by companies, biomedical industries, and other sectors
- Features at least 50% of references from the last 2-3 years
Frequently asked questions
At the moment all of our mobile-responsive ePub books are available to download via the app. Most of our PDFs are also available to download and we're working on making the final remaining ones downloadable now. Learn more here.
Both plans give you full access to the library and all of Perlegoâs features. The only differences are the price and subscription period: With the annual plan youâll save around 30% compared to 12 months on the monthly plan.
We are an online textbook subscription service, where you can get access to an entire online library for less than the price of a single book per month. With over 1 million books across 1000+ topics, weâve got you covered! Learn more here.
Look out for the read-aloud symbol on your next book to see if you can listen to it. The read-aloud tool reads text aloud for you, highlighting the text as it is being read. You can pause it, speed it up and slow it down. Learn more here.
Yes, you can access Materials for Biomedical Engineering: Biopolymer Fibers by Valentina Grumezescu,Alexandru Grumezescu in PDF and/or ePUB format, as well as other popular books in Technology & Engineering & Materials Science. We have over one million books available in our catalogue for you to explore.
Information
Chapter 1
Polymer fibers in biomedical engineering
Alexandra Nicolae1 and Alexandru Mihai Grumezescu2,3, 1Faculty of Foreign Languages, University Politehnica of Bucharest, Bucharest, Romania, 2Faculty of Applied Chemistry and Materials Science, University Politehnica of Bucharest, Bucharest, Romania, 3ICUBâResearch Institute of University of Bucharest, Bucharest, Romania
Abstract
Polymer fibers are intensively used in biomedical engineering because of similarity with the extracellular matrix and due to their versatility. The methods of obtaining polymer fibers are diverse. Natural polymers, such as collagen, silk, and cellulose, can be used to fabricate a fiber network in which cells can attach and proliferate. Polymeric synthetic are used for reinforcement and to offer mechanical support. Electro-spinning is the main method to obtain a fibrous biomaterial. Phase separation, self-assembly, or templating is also used to fabricate polymeric material. The applications in biomedical engineering are essentially for human health. Burns, fractures, cartilage, and muscle disease are problems that medicine is still trying to solve and that can be treated faster with biomaterials. The versatility of these medical devices led to the development of systems that can facilitate the remodulation of injured tissue.
Keywords
Polymer fibers; biomedical engineering; electro-spinning
1.1 Introduction
In recent years, medical research has evolved considerably with the aim of identifying innovative methods of treating various diseases. The most common applications of biomaterials have been reported in ophthalmology and in dentistry, but recently their use in cell biology, microbiology, engineering, and material science has gotten the attention of researchers (Keane and Badylak, 2014).
Biomaterials are designed to replace or restore tissue function (Appel et al., 2013) in order to improve the activity of the damaged biological structure. Currently, all types of materials, metallic, polymeric, ceramic, or composite, are used in medicine. The need for these materials has increased with the increasingly detailed study of physical processes. Thus biomaterials must meet complex requirements to not alter existing structures where they will be introduced.
In the human body there is a considerable amount of protein, enzymes, and polysaccharides. All of which are part of the polymerâs category, so, in biomedical applications, polymeric materials are used in a high percentage in both natural and synthetic forms. For obtaining a medical device, these materials must possess biocompatibility, be nontoxic, provide the necessary mechanical support to the injured area, allow cell development, and not cause inflammation.
The reasons polymers are one of the best choices for biomaterials include their availability, the facility with which they are obtained, their versatile properties that can be adjusted for a particular function and the wide range of applications in which they can be used (Ozdil and Aydin, 2014).
Polymer fibers have superior advantages in biomedical engineering, which is why they are heavily used in numerous applications (Mohammed et al., 2015). Due to the improved properties, these fibers can be better integrated in the human body ensuring a high similarity with the native extracellular matrix (Liu, H. et al., 2013). The variability of the form in which polymers may exist along with the enhancements from fiber reinforcement (Parandoush and Lin, 2017) has led to promising materials with biomedical applications. Natural or synthetic fibers (Li, X. et al., 2014), these scaffolds can interact differently with the biological structures depending on their size, form, or time of action. Also, the orientation of fibers is an important property. This aspect could change the bodyâs response when in contact with the damaged tissues (Li, X. et al., 2014).
The evolution of biomedical engineering has exposed over time the appearance of materials with superior properties and the identification of innovative methods of obtaining them. In choosing the technological process of obtaining polymers fibers, it is important to ensure good interaction with the tissue and to improve the healing time of the affected sites. Today, the use of these polymeric biomaterials is necessary in a lot of medical applications because they are some of the best tolerated medical devices by the human body.
1.2 Methods for Obtaining Polymer Fibers
1.2.1 Materials to Fabricate Polymers Fibers
An important feature in terms of polymeric fibers is the interaction with biological systems. It is essential that the materials do not produce undesirable changes in the human body. The materials used for obtaining polymer fibers can come from natural sources or can be synthetic (Kundu et al., 2014).
1.2.1.1 Natural polymers
Sources for natural polymers are diverse. As far as the biomaterial field is concerned, the polymers are extracted from microorganisms, animals, and plants. Natural polymers, due to the benefits they offer, are a good alternative in fiber manufacturing. The main advantages of them are the similarity to host tissue, the fast integration into the injured site, the biocompatibility, the biodegradation, and the possibility of being degraded by enzymes and the ability to react with different biological structures (Asghari et al., 2017). Currently, there are a high number of natural polymers that can be processed through advanced technologies with the goal of obtaining fibrillar structures.
Collagen, an important protein of the extracellular matrix, has an increased interest in regenerative medicine. Over time, this polymer has been used in a lot of applications, and is currently one of the most extensively studied biomaterials (Jha et al., 2011). In most tissues, collagen fibrils and the networks (Chattopadhyay and Raines, 2014b) are designed to compact the extracellular matrix and provide cell protection. Biomaterials based on collagen have some amazing properties. They are biocompatible, biodegradable, do not produce inflammation, cause faster healing, and succeed in forming links with surrounding structures (Asghari et al., 2017; PÄunica-Panea et al., 2016).
Stable collagen fibers can be obtained by cross-linking and self-aggregation (Chattopadhyay and Raines, 2014b). As a material in biomedical engineering, these polymer fibers can form a scaffold that can be useful in the process of regeneration of a tissue. Collagen flexibility led to the possibility to process it in various forms such as sponges, sheets, tubes, foams, and nanofibers for biomedical engineering (Law et al., 2016). This advantage allows achieving performance in a number of branches of medicine.
Another natural polymer that researchers have focused on lately is cellulose. Cellulose is a natural homopolymer with high molecular weight that has 1,4-linked anhydro D-glucose unites. This polymer is found frequently in nature, and is present in wood, cotton, plants, and even in marine animals (Halib et al., 2017).
Cellulose nanofibers are based on microfibrils, and these nanomaterials have a structure both crystalline and amorphous, compared with other forms reported in the literature. The nanofibers can be extracted and purified from plant fibers by various methods, such as mechanical treatments, chemical treatments, or a combination of both treatments (Chakrabarty and Teramoto, 2018; Abitbol et al., 2016). Due to being nanometer in size and having good biologic properties, the cellulose nanofibers are intensively used in biomedical engineering. With high biocompatibility, nontoxicity, biodegradability, and a high degree of reinforcement (Dufresne, 2013), this polymer has incredible potential in medical applications such as tissue engineering, wound healing, cartilage regeneration, and dentistry (Halib et al., 2017).
Cellulose fiber efficacy has also been demonstrated in the production of composites. The nanocomposites are intensively used due to their superior properties. According to Liu, D. et al. (2013), a composite based on cellulose fiber-reinforced polyvinyl alcohol, a synthetic polymer, was obtained. The study showed an improvement in mechanical properties by adding these polymer fibers. Also, the thermal stability and transparency of the processed biomaterial were within optimal limits, thus increasing its potential in medical and optical applications (Liu, D. et al., 2013).
Silk fibers, another natural biomaterial that has gotten the attention of researchers, are used in medical applications. Silk fibers have properties that ensure biocompatibility, limited pathogen transmission, biodegradation, nontoxicity, and a very good support through their mechanical property. The scaffolds based on silk can be obtained by various technologies, with the applied parameters giving variety in the shape and size of the fibers (Ma et al., 2018). Fine fibers, with a reduced size from micrometers to tens of nanometers, have recently been synthesized with the aim of providing cellular support in tissue engineering. This study determined the influence of the environment in which the fibers were obtained and established an optimal mechanical characteristic for this scaffold synthesized in an aqueous solution. The results may encourage the use of silk fibers in medical applications where the solvent is an important factor for the human cells (Kishimoto et al., 2017).
In addition to these polymers, there are many other ways to obtain polymer fibers. Chitosan, pectin, and agarose are some examples of materials with promising properties for biomedical engineering.
1.2.1.2 Synthetic polymers
Synthetic polymers can also be used for fiber manufacturing. This type of polymer has the advantages of providing better mechanical support than natural polymers and is cheaper. Synthetic polymers are an optimal choice to reinforce materials based on natural substances.
Poly(Δ-caprolactone) is a aliphatic polyester. As a biocompatible and biodegradable polymer, it is successfully used in plastic surgery or in drug-delivery systems. Poly(Δ-caprolactone) has a lot of benefits such as compatibility with another polymers, but also with biological structures, can be easy to process, can exist in various forms, is low cost, and is biodegradable which can improve patient recovery by stimulating the body to heal itself (Azimi et al., 2014).
Composites based on poly(Δ-caprolactone) have shown better results by adding different materials. For example, in a study about bone regeneration, Wang et al. (2010) used a composite that contained poly(L-lactide-co-Δ-caprolactone), polyethylene oxide, and hydroxyapatite to produce a nanofibrous membrane with the aim of improving bone restoration....
Table of contents
- Cover image
- Title page
- Table of Contents
- Copyright
- List of Contributors
- Series Preface
- Preface
- Chapter 1. Polymer fibers in biomedical engineering
- Chapter 2. Organicâinorganic micro/nanofiber composites for biomedical applications
- Chapter 3. Polymer fiber-based biocomposites for medical sensing applications
- Chapter 4. Nanocomposite electrospun micro/nanofibers for biomedical applications
- Chapter 5. âGreenâ polymeric electrospun fibers based on tree-gum hydrocolloids: Fabrication, characterization and applications
- Chapter 6. Aramid fibers composites to innovative sustainable materials for biomedical applications
- Chapter 7. Bio-based nanocomposites: Strategies for cellulose functionalization and tissue affinity studies
- Chapter 8. Polyethersulfone fiber
- Chapter 9. Spider silk fibers: Synthesis, characterization, and related biomedical applications
- Chapter 10. Silk fiber composites in biomedical applications
- Chapter 11. Silk and silk fibroin-based biopolymeric composites and their biomedical applications
- Chapter 12. Keratin-based matrices from wool fibers and human hair
- Chapter 13. Polyhydroxybutyrate: Development and applications as a biodegradable biotextile
- Index