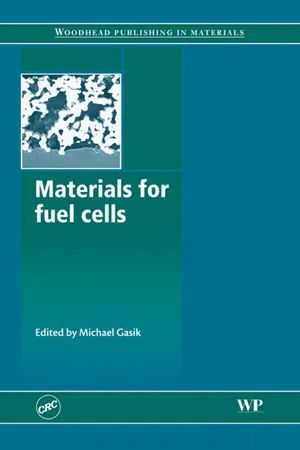
- 512 pages
- English
- ePUB (mobile friendly)
- Available on iOS & Android
Materials for Fuel Cells
About This Book
A fuel cell is an electrochemical device that converts the chemical energy of a reaction (between fuel and oxidant) directly into electricity. Given their efficiency and low emissions, fuel cells provide an important alternative to power produced from fossil fuels. A major challenge in their use is the need for better materials to make fuel cells cost-effective and more durable. This important book reviews developments in materials to fulfil the potential of fuel cells as a major power source.After introductory chapters on the key issues in fuel cell materials research, the book reviews the major types of fuel cell. These include alkaline fuel cells, polymer electrolyte fuel cells, direct methanol fuel cells, phosphoric acid fuel cells, molten carbonate fuel cells, solid oxide fuel cells and regenerative fuel cells. The book concludes with reviews of novel fuel cell materials, ways of analysing performance and issues affecting recyclability and life cycle assessment.With its distinguished editor and international team of contributors, Materials for fuel cells is a valuable reference for all those researching, manufacturing and using fuel cells in such areas as automotive engineering.
- Examines the key issues in fuel cell materials research
- Reviews the major types of fuel cells such as direct methanol and regenerative fuel cells
- Further chapters explore ways of analysing performance and issues affecting recyclability and life cycle assessment
Frequently asked questions
Information
Introduction: materials challenges in fuel cells
Publisher Summary
1.1 What is a fuel cell?
1.2 Why fuel cells?
1.3 Which fuel cell?
1.4 Fuel cells and materials challenges
Table of contents
- Cover image
- Title page
- Table of Contents
- Related titles
- Copyright
- Contributor contact details
- Chapter 1: Introduction: materials challenges in fuel cells
- Chapter 2: Materials basics for fuel cells
- Chapter 3: Alkaline fuel cells
- Chapter 4: Polymer electrolyte membrane fuel cells
- Chapter 5: Direct methanol fuel cells
- Chapter 6: Phosphoric acid fuel cells
- Chapter 7: Molten carbonate fuel cells
- Chapter 8: Solid oxide fuel cells
- Chapter 9: Regenerative fuel cells
- Chapter 10: Novel fuel cells and materials
- Chapter 11: Performance degradation and failure mechanisms of fuel cell materials
- Chapter 12: Recycling and life cycle assessment of fuel cell materials
- Index