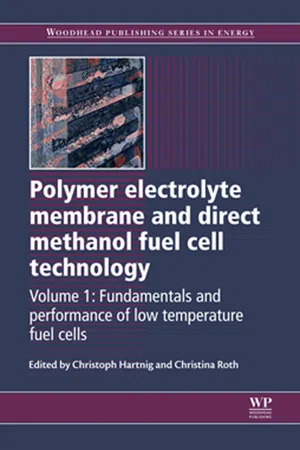
Polymer Electrolyte Membrane and Direct Methanol Fuel Cell Technology
Volume 1: Fundamentals and Performance of Low Temperature Fuel Cells
- 430 pages
- English
- ePUB (mobile friendly)
- Available on iOS & Android
Polymer Electrolyte Membrane and Direct Methanol Fuel Cell Technology
Volume 1: Fundamentals and Performance of Low Temperature Fuel Cells
About This Book
Polymer electrolyte membrane fuel cells (PEMFCs) and direct methanol fuel cells (DMFCs) technology are promising forms of low-temperature electrochemical power conversion technologies that operate on hydrogen and methanol respectively. Featuring high electrical efficiency and low operational emissions, they have attracted intense worldwide commercialization research and development efforts. These R&D efforts include a major drive towards improving materials performance, fuel cell operation and durability. In situ characterization is essential to improving performance and extending operational lifetime through providing information necessary to understand how fuel cell materials perform under operational loads.This two volume set reviews the fundamentals, performance, and in situ characterization of PEMFCs and DMFCs. Volume 1 covers the fundamental science and engineering of these low temperature fuel cells, focusing on understanding and improving performance and operation. Part one reviews systems fundamentals, ranging from fuels and fuel processing, to the development of membrane and catalyst materials and technology, and gas diffusion media and flowfields, as well as life cycle aspects and modelling approaches. Part two details performance issues relevant to fuel cell operation and durability, such as catalyst ageing, materials degradation and durability testing, and goes on to review advanced transport simulation approaches, degradation modelling and experimental monitoring techniques.With its international team of expert contributors, Polymer electrolyte membrane and direct methanol fuel cell technology Volumes 1 & 2 is an invaluable reference for low temperature fuel cell designers and manufacturers, as well as materials science and electrochemistry researchers and academics.
- Covers the fundamental science and engineering of polymer electrolyte membrane fuel cells (PEMFCs) and direct methanol fuel cells (DMFCs), focusing on understanding and improving performance and operation
- Reviews systems fundamentals, ranging from fuels and fuel processing, to the development of membrane and catalyst materials and technology, and gas diffusion media and flowfields, as well as life cycle aspects and modelling approaches
- Details performance issues relevant to fuel cell operation and durability, such as catalyst ageing, materials degradation and durability testing, and reviews advanced transport simulation approaches, degradation modelling and experimental monitoring techniques
Frequently asked questions
Information
Fuels and fuel processing for low temperature fuel cells
Abstract:
1.1 Introduction
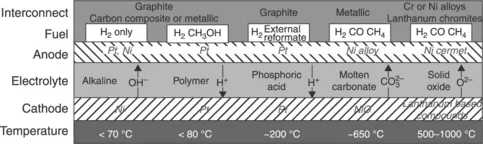
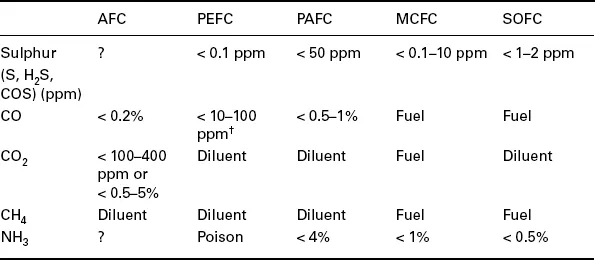
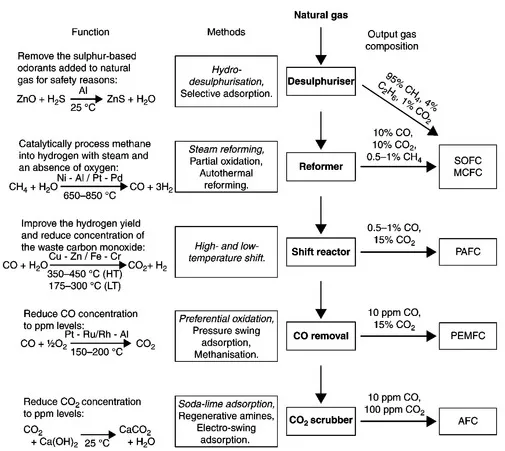
1.2 Thermodynamics of fuel cell operation and the effect of fuel on performance
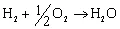




Table of contents
- Cover image
- Title page
- Table of Contents
- Copyright
- Contributor contact details
- Woodhead Publishing Series in Energy
- Preface
- Part I: Fundamentals of polymer electrolyte membrane and direct methanol fuel cell technology
- Part II: Performance issues in polymer electrolyte membrane and direct methanol fuel cells
- Index