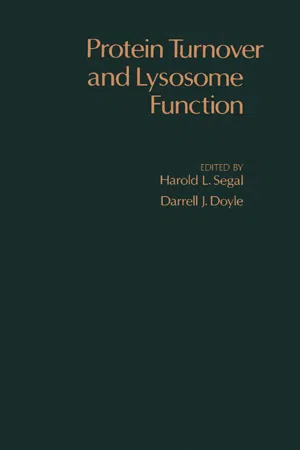
This is a test
- 810 pages
- English
- ePUB (mobile friendly)
- Available on iOS & Android
eBook - ePub
Protein Turnover and Lysosome Function
Book details
Book preview
Table of contents
Citations
About This Book
Protein Turnover and Lysosome Function comprises the proceedings of a symposium under the same title held at the State University of New York at Buffalo on August 21-26, 1977. The book discusses mechanisms of protein turnover, as well as the identification and characterization of intracellular proteases. The text also describes the internalization of macromolecules into the intracellular digestive system; the types of specificity entailed; and the fate of the membrane material involved in the vacuolization process. Biochemists, pathologists, cell biologists, molecular biologists, and physiologists will find the book invaluable.
Frequently asked questions
At the moment all of our mobile-responsive ePub books are available to download via the app. Most of our PDFs are also available to download and we're working on making the final remaining ones downloadable now. Learn more here.
Both plans give you full access to the library and all of Perlego’s features. The only differences are the price and subscription period: With the annual plan you’ll save around 30% compared to 12 months on the monthly plan.
We are an online textbook subscription service, where you can get access to an entire online library for less than the price of a single book per month. With over 1 million books across 1000+ topics, we’ve got you covered! Learn more here.
Look out for the read-aloud symbol on your next book to see if you can listen to it. The read-aloud tool reads text aloud for you, highlighting the text as it is being read. You can pause it, speed it up and slow it down. Learn more here.
Yes, you can access Protein Turnover and Lysosome Function by Harold L. Segal,Darrell J. Doyle in PDF and/or ePUB format, as well as other popular books in Scienze biologiche & Biologia. We have over one million books available in our catalogue for you to explore.
Information
Topic
Scienze biologicheSubtopic
BiologiaINTRODUCTION
A number of aspects of the mechanism and regulation of protein turnover, many of which are dealt with in the succeeding papers in this volume, may be illustrated by reference to the translocations and transformations illustrated in Fig. 1.
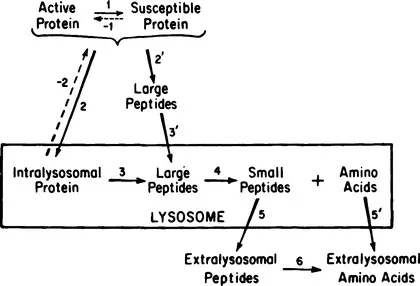
In this schematic diagram are traced the possible pathways of degradation of an intact protein to its constituent amino acids. Two alternatives exist at the beginning. One entails uptake of the protein into the digestive compartment prior to the first degradative step (steps 2 and 3), and the other the inverse (steps 2’ and 3’).
Whichever precedes, uptake or proteolysis, a prior conversion of the protein to a susceptible form may be involved (step 1). The dashed arrow is meant to imply that this reaction may be reversible or irreversible. The latter type may entail denaturation, or a marking for destruction by a covalently added or removed tag, such as a phosphate, glycosyl, acetyl, or methyl group. Interesting recent examples have come from the work of Ashwell and Morell (1), in which it was shown that removal of sialic acid from certain circulating glycoproteins greatly increased their rate of uptake and ultimate digestion by the liver. Subsequent removal of some of the underlying galactose residues eliminated the rapid uptake. One may surmise that desialyation may be a physiological process and the rate-limiting step in the turnover of these serum glycoproteins.
Certain aspects of the degradation of these desialyated proteins by isolated hepatocytes are discussed by Berg (this volume). Other aspects of protein turnover in isolated hepatocytes are discussed by Wagle et al. (this volume). The use of isolated hepatocytes as a system for the study of protein turnover more amenable to experimental manipulation than the in situ rat liver is discussed by Seglen (this volume).
Reversible formation of a susceptible form of a protein may also imply that one of the equilibrium conformational states in which proteins are known to exist is the one susceptible to uptake or proteolytic attack, or may represent association or disassociation of a cofactor or other ligand. Examples of such cases with possible physiological relevance are the stabilization of phosphofructokinase by a peptide factor isolated from liver and the stabilization of arginase by Mn2+ or amino acids, discussed by Segal et al. (this volume). However, with other enzymes including alanine aminotransferase and tyrosine aminotransferase, coenzyme dissociation is not a significant determinant of the in vivo rate, according to the findings of Kenney and Lee (this volume). Rather, in the view of these workers, the cofactor dissociation rate and the intracellular stability may reflect structural features of the proteins that determine both properties.
Uptake of intact proteins (step 2) may represent a site of selectivity in turnover rates, whether uptake of extracellular or intracellular proteins is involved. Such selectivity implies a recognition capability, either broad or specific. Specific receptors on the external surface of the plasma membrane have been identified in the laboratories of Stahl (this volume) and Sly (this volume) for certain lysosomal enzymes that find their way into the circulation, and by Brown and Goldstein (this volume) for serum lipoproteins. Similarly, Michl and Silverstein (this volume) discuss the specific inhibition of FC and C3 plasma membrane receptor-mediated phagocytosis by 2-deoxy-D-glucose.
A broader specificity based on lipophilic affinities has been suggested by work of Dean (2, this volume), Bohley (this volume), and Segal et al. (this volume). In addition, Segal et al. have recently shown a general regulation of pinocytic uptake, using the rat yolk sac as a model system. The development and further properties of the rat yolk sac as a system for studying protein degradation are discussed by Lloyd and Williams (this volume).
The fates of both the interiorized protein and the unit of membrane involved in the uptake and recognition of extracellular proteins are discussed by several workers in this volume, including Brown et al. Doyle, and Kosmakos. Similarly, Atkinson (this volume) discusses the overall pathway of synthesis and degradation of membrane glycoproteins and other proteins using vesicular stomatitis virus-infected HeLa cells as a model system.
A major problem in understanding the uptake step is the disposition of the membrane unit of the endocytosed or autophagic vacuole after delivery of the contents to the lysosome. The rates of commitment of membrane material for the formation of such vacuoles greatly exceed the rates of membrane renewal, suggesting that recycling must occur. The work of Doyle (this volume), Reutter (this volume), and Tulkens (this volume) on membrane and vacuole formation is relevant to this aspect of the process.
Additional evidence implicating the lysosomes as the intracellular site for the degradation of exogenous proteins comes from the studies of Poole and Warburton (this volume), who used chloroquine to inhibit lysosomal function of macrophages and fibroblasts. In a similar approach utilizing chloroquine and other lysosomotropic drugs, Strauss and Flickinger (this volume) present evidence implicating a role for lysosomal degradation in corpus luteum function.
Strong evidence implicating the lysosome as a site for the degradation of at least some of the endogenous cell protein, particularly during nutritional step-down conditions, comes from the studies of Mortimore (this volume) using the perfused liver system. Data suggesting different mechanisms for basal degradation, perhaps not involving lysosomes, versus the lysosomal-type degradation occurring in nutritional step-down conditions are given by Poole and Warburton (this volume) and by Dice and Walker (this volume). The importance of protein degradation in the regulation of liver growth is discussed by Scornik (this volume), who proposes that the effect of growth on degradation is not peculiar to this physiological state but is a general one.
Selectivity in susceptibility of intact proteins to lysosomal proteases (step 3) has also been shown by the work of Bohley et al. (3, this volume), Dean (4, this volume), and Segal et al. (this volume). Furthermore, the proteolytic susceptibility among a population of proteins was in accord with their in vivo turnover rates. The nature and characterization of the lysosomal enzymes capable of degrading a vareity of cellular macromolecules is discussed by Barrett (this volume), Touster (this volume), Turk (this volume), and Kalnitsky (this volume), while Swank (this volume) describes experiments concerned with understanding the biogenesis of lysosomal enzymes themselves, using a genetic system as a tool to complement biochemical analysis. Schneider and Cornell (this volume) present evidence for the existence of an ATP-driven proton pump in lysosomes, which could function to provide the acid pH that is optimum for most lysosomal hydrolases.
However, a conceptual difficulty in attributing selectivity to the intralysosomal proteolytic step is that it implies a reversibility of previous steps, notably the uptake step. Some observations on the disposition of macromolecular markers subsequent to their uptake by the liver are consistent with a release from the lysosomal compartment, but the data are not yet sufficient to exclude other interpretations (Segal et al., this volume).
Limited extralysosomal proteolysis has been shown to occur by Katunuma’s laboratory for certain apopyroxidal proteins (5), and by Horecker with fructose bisphosphatase (this volume). In addition, Goll (this volume), Waxman and Krebs (this volume), Stracher (this volume), Bird (this volume), and Bhan and Hatcher (this volume) have studied nonlysosomal proteases of muscle which may be involved in the turnover of the myofibril in this tissue. The turnover rates of the myofibril proteins myosin and actin vary as a function of the physiological state of the cells, as reported by Nihei (this volume). Actually, total protein turnover in muscle varies markedly in a variety of dietary and hormonal growth conditions, as shown by the studies of Millward (this volume). Furthermore, the changes in muscle protein breakdown in anabolic or catabolic states are often opposite to those expected, suggesting that synthesis of muscle proteins also must undergo marked changes during adaptation. In this context, Lebherz et al. (this volume) studied the differential regulation of glycolytic enzymes in red and white muscle, which is primarily at the level of synthesis.
It also appears from work done in the laboratories of Goldberg (this volume) and of Hershko (this volume) that a nonlysosomal protease is involved in the selective degradation of abnormal proteins of the reticulocyte. Little is known about the normal degradation of the major erythrocyte proteins, but the turnover characteristics of the different globin chains are discussed by Garrick et al. (this volume).
Using another system, less complex than the animal cell, Holzer (this volume) discusses the control of proteolysis in yeast. In this system five different proteinases have been localized to yeast vacuoles. The activity of these proteinases may be controlled by one or more of three classes of inhibitor in the cytosol, demonstrating that even in a simple eukaryote the regulation of proteolysis is complex, possibly involving cascade-type mechanisms.
Although the first proteolytic step, wherever it occurs, would be expected to be irreversible and to commit the protein irrevocably to total hydrolysis, it need not necessarily lead to loss of function. It has been shown, for example, by Horecker (this volume) that an active species remains after proteolytic modification of fructose bisphosphatase, and the same is true for ribonuclease (6), ATP citrate lyase (7), and mung bean nuclease (8). Evidence obtained in the laboratory of Segal (this volume) suggests it may also be true for invertase subsequent to its ingestion by liver cells.
The development of new techniques and concepts such as highly resolving two-dimensional methods of separating proteins used by Larrabee (this volume), radioactive labeling of proteins to high specific activity by the method of Pine and Schimke (this volume), a better understanding of compartmentation and precursor pools as discussed by Khairallah (this volume), and the use of lipid vesicles to introduce specific macromolecules into animal ce...
Table of contents
- Cover image
- Title page
- Table of Contents
- Inside Front Cover
- Copyright
- List of Contributors
- Preface
- Chapter 1: INTRODUCTION
- MECHANISMS AND REGULATION OF PROTEIN TURNOVER
- LYSOSOMAL AND NONLYSOSOMAL PROTEASES
- UPTAKE OF PROTEINS INTO THE LYSOSOMAL SYSTEM
- PROTEIN TURNOVER IN MUSCLE AND HEART
- TURNOVER OF MEMBRANE PROTEINS