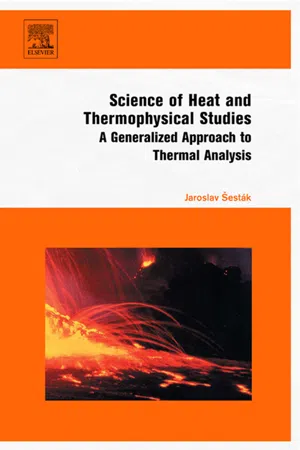
eBook - ePub
Science of Heat and Thermophysical Studies
A Generalized Approach to Thermal Analysis
This is a test
- 486 pages
- English
- ePUB (mobile friendly)
- Available on iOS & Android
eBook - ePub
Science of Heat and Thermophysical Studies
A Generalized Approach to Thermal Analysis
Book details
Book preview
Table of contents
Citations
About This Book
Science of Heat and Thermophysical Studies provides a non-traditional bridging of historical, philosophical, societal and scientific aspects of heat with a comprehensive approach to the field of generalized thermodynamics. It involves Greek philosophical views and their impact on the development of contemporary ideas. Covered topics include:
• the concept of heat • thermometry and calorimetry • early concepts of temperature and its gradients • non-equilibrium and quantum thermodynamics • chemical kinetics • entropy, order and information • thermal science applied to economy(econophysics), ecosystems, and process dynamics or mesoscopic scales (quantum diffusion) • importance of energy science and its influence to societal life
Frequently asked questions
At the moment all of our mobile-responsive ePub books are available to download via the app. Most of our PDFs are also available to download and we're working on making the final remaining ones downloadable now. Learn more here.
Both plans give you full access to the library and all of Perlego’s features. The only differences are the price and subscription period: With the annual plan you’ll save around 30% compared to 12 months on the monthly plan.
We are an online textbook subscription service, where you can get access to an entire online library for less than the price of a single book per month. With over 1 million books across 1000+ topics, we’ve got you covered! Learn more here.
Look out for the read-aloud symbol on your next book to see if you can listen to it. The read-aloud tool reads text aloud for you, highlighting the text as it is being read. You can pause it, speed it up and slow it down. Learn more here.
Yes, you can access Science of Heat and Thermophysical Studies by Jaroslav Sestak in PDF and/or ePUB format, as well as other popular books in Sciences physiques & Chimie physique et théorique. We have over one million books available in our catalogue for you to explore.
Information
Topic
Sciences physiquesSubtopic
Chimie physique et théoriqueChapter 1
SOME PHILOSOPHICAL ASPECTS OF SCIENTIFIC RESEARCH
1.1. Exploring the environment and scale dimensions
The understanding of nature and its description are not given a priori but developed over time, according to how they were gradually encountered and assimilated into man’s existing practices. Our picture of nature has been conditioned by the development of modes of perceiving (sensors) and their interconnections during mankind’s manufacturing and conceptual activities. The evaluation of sensations required the definition of measuring values, i.e., the discretion of what is available (experience, awareness, inheritance). A sensation must be classified according the given state of the organism, i.e., connected to the environment in which the evaluation is made. Everyday occurrences have been incorporated, resulting in the outgrowth of so-called custom states. This is, however, somewhat subjective because for the sake of objectivity we must develop measures independent of individual sensation, i.e., scales for identifying the conceptual dimensions of our surroundings (territorial and/or force-field parameters such as remoteness (distance) or warmth (temperature), having mutually quite irreconcilable characteristics).
Our educational experience causes most of us to feel like inhabitants of a certain geographical (three-dimensional) continuum in which our actual position or location is not necessarily indispensable. A similar view may be also applied to the other areas such as knowledge, durability, warmth etc. If we were, for example, to traverse an arbitrary (assumingly 2-D) landscape we would realize that some areas are more relevant than others. Indeed, the relative significance of acknowledged objects depends on their separated distance – which can be described as their ‘nearness’ [17]. It can be visualized as a function, the value of which proportionally decreases with the distance it is away from us, ultimately diminishing entirely at the ‘horizon’ (and the space beyond). The horizon, as a limit, exists in many of our attributes (knowledge, experience, capability).
When the wanderer strolls from place to place, his ‘here’, ‘there’, his horizon as well as his field of relevance gradually shifts whilst the implicit form of nearness remains unchanged. If the various past fields of relevance are superimposed, a new field of relevance emerges, no longer containing a central position of ‘here’. This new field may be called the cognitive map, as coined by Havel [17] for our positional terrain (as well as for our knowledge extent). Individual cognitive maps are shaped more by memories (experience, learning) of the past than by immediate visual or kinestatic encounters.

Fig. 2 Illustrative zoom as a shift in the scale dimension and right a symbolic communication user who exploits a limited range of scales to its explication [9, 17]. Courtesy of Ivan M. Havel, Prague, Czech Republic
It is not difficult to imagine a multitude of cognitive maps of some aggregates to form, e.g., a collective cognition map of community, field, etc., thus available for a wider public use. However, to match up individual maps we need to be sure of the application of adequately rational levels, called scales [18]. Returning to the above geographical illustrations we may see them as the superimposition of large-scale maps on top of another smaller-scale maps, which, together, yield a more generalized dimension, called the ‘spatial-scale axis’. A movement in the upward direction along this scale axis resembles zooming out using a camera (objects shrink and patterns become denser) while the opposite downward movement is similar to zooming in (objects are magnified and patterns become dispersed or even lost). Somewhere in the center of this region (about the direct proportions one-to-one) exists our perception of the world, a world that, to us, is readily understandable.
Our moving up and down in the scale is usually conditioned by artificial instruments (telescopes, microscopes) that are often tools of scientific research. Even when we look through a microscope we use our natural vision. We do not get closer to the observed but we take the observed closer to us, enabling new horizons to emerge and employ our imagination and experience. We may say that we import objects, from that other reality, closer to us. Only gradually have the physical sciences, on the basis of laborious experimental and theoretical investigations, extended our picture of nature to such neighboring scales.
Let us consider the simplest concept of shape. The most common shapes are squares or circles easily recognizable at a glance – such objects can be called ‘scale-thin’ [17]. Certainly there are objects with more complex shapes such as the recently popular self-similar objects of fractals that can utilize the concept of the scale dimension quite naturally because they represent a recursive scale order. It is worth noting that the term fractal was derived from the Latin word ‘fractus’ (meaning broken or fragmented) or ‘frangere’ (break) and was coined by the Polish-born mathematician Mandelbrot on the basis of Hausdorff dimension analysis. The term fractal dimension reveals precisely the nuances of the shape and the complexity of a given non-Euclidean figure but as the idiom dimension does not have exactly the same meaning of the dimension responsive to Euclidean space so that it may be better seen as a property [9].
Since its introduction in 1975, it has given rise to a new system of geometry, impacting diverse fields of chemistry, biology, physiology and fluid dynamics. Fractals are capable of describing the many irregularly shaped objects or spatially non-uniform phenomena in nature that cannot be accommodated by the components of Euclidean geometry. The reiteration of such irregular details or patterns occur at progressively smaller scales and can, in the case of purely abstract entities, continue indefinitely so that each part of each part will look basically like the object as a whole. At its limit, some ‘conclusive’ fractal structures penetrate through arbitrary small scales, as its scale relevance function does not diminish as one zooms up and down. It reflects a decision by modern physics to give up the assumption of the scale invariance (e.g., different behavior of quantum and macroscopic particles).
Accordingly, the focus became the study of properties of various interfaces, which are understood as a continuity defect at the boundary between two entities regardless of whether it is physics (body surface, phase interfaces), concepts (classical and quantum physics, classical and nonequilibrium thermodynamics), fields of learning (thoughts, science and humanities) or human behavior (minds, physical and cultural frontiers). In our entrenched and customary visualization we portray interfaces only (as a tie line, shed, curve) often not monitoring the entities which are borderlined. Such a projection is important in conveniently picturing our image of the surroundings (models in physics, architectonical design). Interfaces entirely affect the extent of our awareness, beyond which our confusion or misapprehension often starts. As mentioned above, it brings into play an extra correlation, that is the interface between the traditional language of visible forms of the familiar Euclidean geometry and the new language used to describe complex forms often met in nature and called fractals.
The role of mathematics in this translation is important and it is not clear to what extent mathematical and other scientific concepts are really independent of our human scale location and the scale of locality. Vopěnka [19] in 1989 proposed a simplifying program of naturalization of certain parts of mathematics: “we should not be able to gain any insight about the classical (geometrical) world since it is impossible to see this world at all. We see a world bordered on a horizon, which enables us to gain an insight, and these lead us to obtain nontrivial results. However, we are not seeing the classical, but the natural (geometrical) world differing in the classification of its infinity as the form of natural infinity” (alternative theory of semi-sets that are countable but infinite beyond the horizon).
One consequence is the way we fragment real-world entities into several categories [17]: things, events and processes. By things, we typically mean those entities which are separable, with identifiable shapes and size, and which persist in time. Events, on the other hand, have a relatively short duration and are composed of the interactions of several things of various sizes. Processes are, in this last property, similar to events but, like things, have a relatively long duration. However, many other entities may have a transient character such as vortices, flames, clouds, sounds, ceremonies, etc. There is an obvious difference between generic categories and particular entities because a category may be scale-thin in two different ways: generically (atoms, birds, etc.) or individually (geometrical concepts, etc.).
There is an interesting asymmetry with respect to the scale axes [18]; we have a different attitude towards examining events that occur inside things than what we consider exists on their outside. Moreover there are only a few relevant scales for a given object, occasionally separated by gaps. When considering, for example, a steam engine, the most important scale is that of macroscopic machinery while the second relevant scale is set much lower, on a smaller scale, and involves the inspection of molecules whose behavior supports the thermodynamic cycle. Whatever the scale spectrum in the designer’s perspective, there is always one and only one relevant ‘scale-here’ range where the meaning of the object or process is located as meaningful.
In the case of complex objects, there is a close relationship between their distribution over scales and a hierarchy of their structural, functional and describable levels. We tend to assign objects of our concern into structural levels and events as well as processes into functional levels. Obvious differences of individual levels yield different descriptions, different terminology (languages) and eventually different disciplines. Two types of difficulty, however, emerge, one caused by our limited understanding of whether and how distinct levels of a system can directly interact and, the other, related to the communication (language) barriers developed over decades of specialization of scientific disciplines (providing the urgent need for cross-disciplinarity).
One of the first mathematical theories in science that dealt with inter-level interactions was Boltzmann’s statistical physics, which is related to thermodynamics and the study of collective phenomena. It succeeded in eliminating the lower (microscopic) level from the macroscopic laws by decomposing the phase space to what is considered macroscopically relevant subsets and by introducing new concepts, such as the mannered entropy principle. It requested to widely adopt the function of logarithm that was already and perpetually accustomed by nature alone (physiology, psychology). In comparison, another scaled sphere of a natural process can be mentioned here where the gradual evolution of living parts has been matured and completed in the log/log relations, called the allometric dependence.
Another relevant area is the study of order/disorder phenomena, acknowledging that microscopically tiny fluctuations can be somewhat ‘immediately’ amplified to a macroscopic scale. What seems to be a purely random event on one level can appear to be deterministically lawful behavior on some other level. Quantum mechanics may serve as another example where the question of measurement is actually the eminent question of interpreting macroscopic images of the quantum-scale events. Factually we construct ‘things’ on the basis of information, which we may call information transducers.
The humanities, particularly economics, are further fascinating spheres for analysis. However, their evaluation can become more complicated as individual scale-levels may mutually and intermediately interact with each other. Namely any forecasting is disconcerted assuming that any weather prediction cannot change the weather itself while economic prediction activity displays the inevitable dependence of what is being evaluated or forecasted.
Yet another sphere of multilevel interactions is the concept of active information – another reference area worthy of mention. Besides the reciprocal interrelation to ‘entropical’ disorder we can also mention the growth of a civilization’s ability to store and process information, which encompasses at least two different scales. On the one hand, there is the need for a growing ability to deal with entities that become composite and more complicated. On the other hand, there is a necessity to compress information storage into smaller and smaller volumes of space. Human progress in its elaborateness is hinted at by the triangle [14] of his rivalry scales: time, t, information, I, and energy, E.
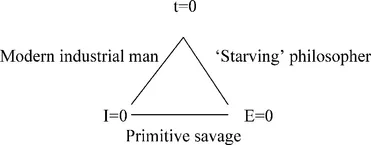
Cyberneticist Weinberg is worth noting as he said “time is likely to become, increasingly, our most important resource. The value of energy and information is, ultimately, that it gives us more freedom to allocate our time”. If we have lots of time we do not need much information because we can indulge in haphazard, slow trial-and-error search. But if time becomes expensive, then we need to know the fastest way to do things and that requires lots of information and time organization. The above treated spatial ‘here’ suggests an obvious analogue in the temporal ‘now’ (temporal field relevance), which is impossible to identify without involving ‘change’ (past and future).
One of the most widespread concepts in various fields (physics, society and/or mind) is the notion of ‘state’ [20]. In fact, there is no any exact physical definition of what is the state alone and we can only assume that a system under consideration must possess its own identity connected to a set of its properties, qualities, internal and external interactions, laws, etc. This ‘identity’ can then be called the state and the description of state is then made upon this set of chosen properties, which must be generally interchangeable when another same system is defined. Thermodynamics (cf. Chapter 6), as one of the most frequent users of the notion of state, is presented as a method for the description and study of various systems, which uses somehow a heterogeneous mixture of abstract variables occasionally defined on different scales, because the thermodynamic concept involves an energetic communication between macro- and microlevels. For example, heat is the transfer of energy to the hidden disordered molecular modes, which makes it troublesome to co-define the packaging value of internal energy when including processes at the macroscopic level, such as the mechanical work (of coordinated molecular motion as whole). Associated with this is the understanding of thermodynamic variables as averaged quantities and the assignment of such variables to individual parts that may be composed together to form other parts [9, 20].
Another important standpoint is the distinction between ‘phase’ as the denomination of a certain intensive state and the different forms of matter (as liquids or solids). In fact, phase keeps traditional meaning as a ‘homogene...
Table of contents
- Cover image
- Title page
- Table of Contents
- Copyright
- Dedication
- PREFACE
- Chapter 1: SOME PHILOSOPHICAL ASPECTS OF SCIENTIFIC RESEARCH
- Chapter 2: MISCELLANEOUS FEATURES OF THERMAL SCIENCE
- Chapter 3: FIRE AS A PHILOSOPHICAL AND ALCHEMICAL ARCHETYPE
- Chapter 4: CONCEPT OF HEAT IN THE RENAISSANCE AND NEW AGE
- Chapter 5: UNDERSTANDING HEAT, TEMPERATURE AND GRADIENTS
- Chapter 6: HEAT, ENTROPY AND INFORMATION
- Chapter 7: THERMODYNAMICS AND THERMOSTATICS
- Chapter 8: THERMODYNAMICS, ECONOPHYSICS, ECOSYSTEMS AND SOCIETAL BEHAVIOR
- Chapter 9: THERMAL PHYSICS OF PROCESS DYNAMICS
- Chapter 10: MODELING THE REACTION MECHANISM: THE USE OF EUCLIDIAN AND FRACTAL GEOMETRY
- Chapter 11: NON-ISOTHERMAL KINETIC BY THERMAL ANALYSIS
- Chapter 12: THERMOMETRY AND CALORIMETRY
- Chapter 13: THERMOPHYSICAL EXAMINATION AND TEMPERATURE CONTROL
- AFTERWORD AND ACKNOWLEDGEMENT
- LITERATURE
- INDEX
- APPENDIX
- About the author