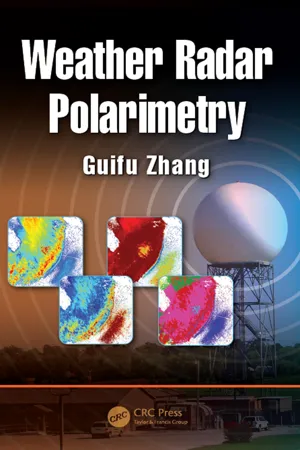
This is a test
- 304 pages
- English
- ePUB (mobile friendly)
- Available on iOS & Android
eBook - ePub
Weather Radar Polarimetry
Book details
Book preview
Table of contents
Citations
About This Book
This book presents the fundamentals of polarimetric radar remote sensing through understanding wave scattering and propagation in geophysical media filled with hydrometers and other objects. The text characterizes the physical, statistical, and electromagnetic properties of hydrometers and establishes the relations between radar observables and physical state parameters. It introduces advanced remote sensing techniques (such as polarimetric phased array radar) and retrieval methods for physical parameters. The book also illustrates applications of polarimetric radar measurements in hydrometer classification, particle size distribution retrievals, microphysical parameterization, and weather quantification and forecast.
Frequently asked questions
At the moment all of our mobile-responsive ePub books are available to download via the app. Most of our PDFs are also available to download and we're working on making the final remaining ones downloadable now. Learn more here.
Both plans give you full access to the library and all of Perlegoâs features. The only differences are the price and subscription period: With the annual plan youâll save around 30% compared to 12 months on the monthly plan.
We are an online textbook subscription service, where you can get access to an entire online library for less than the price of a single book per month. With over 1 million books across 1000+ topics, weâve got you covered! Learn more here.
Look out for the read-aloud symbol on your next book to see if you can listen to it. The read-aloud tool reads text aloud for you, highlighting the text as it is being read. You can pause it, speed it up and slow it down. Learn more here.
Yes, you can access Weather Radar Polarimetry by Guifu Zhang in PDF and/or ePUB format, as well as other popular books in Physical Sciences & Meteorology & Climatology. We have over one million books available in our catalogue for you to explore.
Information
1Â Â Introduction
Radar is an efficient remote sensing system that measures targeted media with four-dimensional information and fine resolution. It plays a critical role in weather observation, detection of hazards, classification and quantification of precipitation, and forecasting. Polarimetric radar provides multiparameter measurements with unprecedented quality and information. In this introduction, the historical development of the weather radar polarimetry is simply summarized, and the motivation and organization of the book are provided.
1.1Â Â HISTORICAL DEVELOPMENT
The history of weather radar development and application is well documented in the first 18 chapters of Radar in Meteorology, edited by Atlas (1990). Here, we briefly summarize the early development and background information that will allow us to progress to weather radar polarimetry.
Radar is an acronym for âradio detecting and ranging of objects,â a remote sensing system that transmits and receives electromagnetic waves to detect and locate targets. Although its history can be traced earlier to the use of radio waves to obtain target information, it was during World War II that radar began to receive a great deal of attention; its technology advanced rapidly in response to the need to detect aircraft. According to Nobel laureate I. I. Rabi, it was radar, not the atomic bomb, that contributed most to the Alliesâ victory in the war.
Prior to the 1930s, the highest radio frequencies used were less than 400 MHz, due to limitations in microwave power generation. The invention of the cavity magnetron made microwave frequency (10 cm and 3 cm wavelength) radars possible and substantially improved detection accuracy and reliability. Once microwave radars were in operation, the marriage between weather and radar occurred naturally. It was immediately observed that rain echoes showed up on the radar displays as clutter, and this clutter sometimes obscured aircraft. One of the early challenges was to remove weather, ground, and sea clutter from radar displays. However, weather measurement in addition to aircraft detection came to be of interest.
At that time, most radar systems were developed by the Radiation Laboratory at the Massachusetts Institute of Technology (MIT). Due to the urgent need for knowledge of radar operation in weather conditions, the Weather Radar Research Project was started in the Department of Meteorology at MIT in 1946. Two radars (10 cm SCT-615 and 3 cm AN/TSP-10) were used to make cloud observations, and their measurements were compared with the direct in situ measurements of hydrometeor particles by instrumented aircraft. A year later, the first radar meteorology conference was held at MIT. D. Atlas was one of ninety attendees. Along with L. Battan, Atlas attended the HarvardâMIT radar school. He then worked at AFCRL (Air Force Cambridge Research Laboratories), where a premier group of scientists and engineers conducted systematic weather radar research in the 1950s. He summarized the early progress in his monograph, Advances in Radar Meteorology, in 1964. L. J. Battan led the Thunderstorm Project at the University of Chicago and completed his PhD work, titled âObservations on Formation of Precipitation in Convective Clouds,â in 1953. Extending his PhD work, in 1959 he wrote Radar Meteorology, the first textbook in the field, with a revision published in 1973. Battan realized that frozen hydrometeors are not spherical, which can cause differences in wave scattering for different polarizations. His book highlighted this fact, with polarization vectors drawn on the cover page.
To address problems of air traffic in the presence of convective weather and to provide timely detection and warning of severe storms, the US Weather Bureau established a national network of 10-cm wavelength radars (WSR-57s). One of the WSR-57s was installed in Norman, Oklahoma, to support research efforts to understand thunderstorms and their effect on the safety of flight. When the National Severe Storms Laboratory (NSSL) was officially formed in Norman in 1962, one of the main objectives was to develop radar technology for severe storm observation. NSSL developed the first 10-cm Doppler weather radar for research to support the National Weather Service and later for polarimetric Doppler radar research. Doppler radar technology was used in the WSR-88D national radar network, which was upgraded with dual-polarization capability by 2013.
The early interaction between NSSL meteorologists and engineers and University of Oklahoma (OU) professors (G. Walker of the EE department and Y. Sasaki of the School of Meteorology) was instrumental in developing the OUâNSSL relationship, which continues to this day. This interaction led to a course in radar meteorology and the textbook Doppler Radar and Weather Observations by Doviak and Zrnic, first published in 1984, with a second edition discussing polarimetry published in 1993. (A paperback Dover edition is now available.) In 1990, an undergraduate textbook was published, Radar for Meteorologists, by Rinehart (1990, 1991, 1997, 2004). While the Doppler technique was a breakthrough for weather observations, radar polarimetry was the next advancement: polarimetric radar data (PRD) with Doppler fields significantly advanced the microphysical and dynamical quantification of clouds and precipitation.
Weather radar polarimetry is defined as the obtaining of weather information through radar measurements with polarization diversity. The major and minor lengths of hydrometeors of various shapes are typically horizontally and vertically oriented, respectively; these different lengths yield differences in wave scattering for different polarization. The polarization of electromagnetic waves is the orientation/direction of the transverse electric field vibrations, which was first explained by Young and Fresnel in the early 1800s. Although polarization had been documented in the Maxwell equations in 1861, weather radar polarimetry did not receive serious consideration until the 1970s. Many works represented and calculated wave polarization and propagation, such as using the PoincarĂ© sphere (PoincarĂ© 1892) and the Stokes parameters (Stokes 1852a, 1852b). The theoretical foundations for wave scattering include the Rayleigh approximation (Rayleigh 1871), Mie theory (Mie 1908), and Gans theory (Gans 1912). The book Light Scattering by Small Particles by Van De Hulst (1957) thoroughly documented these theoretical foundations. Ryde (1941) made the first calculation of hydrometeor scattering and used it to explain the attenuation of radar echoes. Newell and Geotis (1955) summarized earlier studies of meteorological measurements with different polarizations. These studies, which introduced weather radar polarimetry to the radar meteorology community, were mainly focused on the cancellation ratio of power return between circular and linear polarizations and the ratio between the cross-polar and co-polar returns, called the linear depolarization ratio (LDR). Oguchi (1960, 1964, 1983) and Waterman (1965, 1969) successfully made numerical calculations of wave scattering by nonspherical raindrops, which served as the basis for the interpretation of polarimetric data. Doviak and Sirmans (1973) noted the additional information that polarization diversity can provide. McCormick and Hendry (1974) studied the polarization properties of wave propagation in precipitation over a communication link, as well as the power and correlation properties of rain from a polarization diversity radar using circular polarization (McCormick and Hendry 1976). Seliga and Bringi (1976) proposed to improve rain estimation by measuring differential reflectivity (ZDR) between horizontal and vertical polarizations, which received wide attention from the community. The first measurement of differential reflectivity in rain in the âslow-switchedâ mode was successfully made with the CHILL radar during the summer of 1977 and later reported by Seliga et al. (1979). The first âfast-switchedâ ZDR data were obtained in 1978 with the RAL S-band radar in the United Kingdom by Hall et al. (1980). Seliga and Bringi (1978) also proposed that the differential propagation phase could be used to estimate the rain rate, in combination with ZDR.
Largely due to the fast-switched ZDR measurements (Hall et al. 1980), during the 1980s, the weather community in the United States conducted extensive research and implementation of radar polarimetry. The CHILL radar was modified for fast-switched ZDR measurements (Seliga and Bringi 1982). Statistical analysis of measurement error for differential reflectivity was performed by Bringi et al. (1983). At the same time, the differential reflectivity measurement technique was implemented on the CP-2 radar operated by the National Center for Atmospheric Research (NCAR), and differential reflectivity was successfully measured and used for hail detection (Bringi et al. 1984).
Mueller (1984) provided a general formulation for calculating differential propagation phase from a fast-switched polarimetric radar. Meanwhile, NSSL upgraded their Cimarron radar with dual-polarization capabilities and demonstrated on time series data how to obtain Doppler and polarimetric variables on radars with switchable polarization (Sachidananda and ZrniÄ 1985). In addition NSSL scientists and engineers proposed measurements of differential phase ((ÏDP) and co-polar cross-correlation coefficient (Ïhv) (Sachidananda and ZrniÄ 1986, 1989). An additional advance was made when multiparameter radar measurements including LDR were made and used for graupel melting and hail detection studies (Bringi et al. 1986a, 1986b). These early research activities were summarized by Bringi and Hendry (1990) and Seliga et al. (1990).
In the 1990s, research into weather radar polarimetry was extended to the demonstration of the properties of multiparameter polarimetric weather radars. Bringi et al. (1990) examined propagation effects on radar reflectivity measurement. Zahrai and ZrniÄ (1993) demonstrated, for the first time, real-time measurement of full polarimetric radar parameters, including the differential phase, co-polar correlation coefficient, and co-polar and cross-polar correlation coefficients (Ïh and Ïv). At that time, the specific differential phase (KDP), which was the range derivative of ÏDP, received a great deal of attention because it is approximately linearly related to rainfall rate and immune to partial beam blockage and power calibration error (Balakrishnan and ZrniÄ 1990; Ryzhkov and ZrniÄ 1996). A number of polarimetric radar rain estimators were developed based on simulations and studies with simulated or observed raindrop size distributions (DSDs) (Ryzhkov and ZrniÄ 1995; Sachidananda and ZrniÄ 1987; Seliga et al. 1986). Improved quantitative precipitation estimation (QPE) was achieved with real data (Brandes et al. 2002; Giangrande and Ryzhkov 2008; Ryzhkov et al. 2005a, 2005b).
The applications of PRD in cloud and precipitation microphysics studies were further motivated by the success the fuzzy logic technique had in classifying radar echoes (Straka et al. 2000; Vivekanandan et al. 1999; ZrniÄ et al. 2001). A number of field projects that involved S-band polarimetric radar were conducted, including CASES-91 in Kansas, PRECIP98 in Florida (Brandes et al. 2002), and STEPS-00 in Colorado. The data and results from these projects and those from the CSUâCHILL radar and NSSLâs Cimarron and KOUN (NSSLâs R&D WSR-88D upgraded to have polarimetric capability) radars further justified the value of multiparameter PRD in weather service and the need for a dual-polarization upgrade of the national network of NEXRAD radars (Doviak et al. 2000). The book Polarimetric Doppler Weather Radar: Principles and Application was published in 2001, authored by Bringi and Chandrasekar.
To improve model microphysics parameterization and weather forecast, DSDs were retrieved from PRD (Brandes et al. 2004a; Bringi et al. 2002; Cao et al. 2008, 2010; Zhang et al. 2001). A more rigorous way to retrieve microphysics states and to improve quantitative precipitation forecast (QPF) using PRD is to combine them with a numerical weather prediction (NWP) model through data assimilation (Jung et al. 2008a, 2008b). Jung et al. (2008a, 2008b) showed that the addition of PRD (ZDR or KDP) has a positive impact in determining state variables. The polarimetric radar signatures of severe storms were realistically generated from NWP model simulation (Jung et al. 2010a, 2010b; Xue et al. 2010). Radar polarimetryâs greatest potential lies in its ability to accurately retrieve model states and microphysical parameters, which can be used to improve weather prediction. This book is meant to lay a foundation for the efficient and optimal use of PRD for weather quantification and forecasting.
1.2Â Â OBJECTIVES AND ORGANIZATION OF THE BOOK
This book provides the fundamentals and principles of weather radar polarimetry by exploring wave scattering and propagation in cloud and precipitation conditions. The purpose and objectives of this book are illustrated in Figure 1.1. The relations between physical state parameters and radar observables are established so that readers understand the polarimetric radar signatures of typical weather conditions. Advanced signal processing and retrieval algorithms are briefly discussed to help readers better interpret PRD. Data analysis tools and methods are provided for readers to get hands-on experience in dealing with real data.
This book starts with the characterization and representation of hydrometeors in Chapter 2. The physical, statistical, and electromagnetic properties of raindrops, snowflakes, hailstones, and cloud particles are described. The modeling and representation of particle size distribution, shape, and density/composition are presented. Calculations of dielectric constants are included using the Debye model for water and ice and the Maxwell-Garnett and the Polderâvan Santern mixing formulas for airâiceâwater mixtures.

FIGURE 1.1 Sketch of the purpose and objectives of the book.
Chapter 3 provides the fundamentals and calculations of electromagnetic wave scattering from a single particle, which can be a raindrop, snowflake, hailstone, or cloud particle. The scattering representations are included for both spherical and non-spherical particles. The Rayleigh scattering approximation and Mie theory are introduced. In addition, the T-matrix method and its results for hydrometeor scattering calculations are provided.
Chapter 4 describes wave scattering and propagation in clouds and precipitation composed of randomly distributed particles. Coherent and incoherent scattering, as well as their applications, are explained. The wave statistics are illustrated in terms of mean, covariance, and probability density function and linked to polarimetric radar variables. The propagation effects of attenuation, differential attenuation, propagation phase, and differential phase are also included.
Chapter 5 presents signal processing methods for obtaining and improving polarimetric radar measurements, as well as error quantification. Advanced signal processing for improving data quality is introduced, including multilag processing, dual-polarization, and/or dual-scan clutter detection and mitigation.
Chapter 6 documents conventional uses of weather radar polarimetry and shows how cloud and precipitation microphysics can be studied qualitatively and quantitatively. Furthermore, the polarization signatures of typical weather events are presented. The fuzzy logic method for hydrometeor classification is described. QPE and constrained methods for DSD retrieval, as well as attenuation correction, are discussed.
Chapter 7 introduces advanced methods to optimally utilize PRD for QPE and QPF. It describes simultaneous attenuation correction and DSD retrieval, statistical retrieval of rain DSDs, variational analysis, and the challenges and potential of assimilating PRD into NWP models to improve weather forecast.
Chapter 8 discusses phased array weather radar polarimetry that is under development. This includes the theoretical formulation of the phased array polarimetry and the issues, challenges, and possible solutions in designing and developing polarimetric phased array radars for fast date update and accurate weather measurements in future.
2 Characterization of Hydrometeors
The characteristics of polarim...
Table of contents
- Cover
- Half Title
- Title Page
- Copyright Page
- Table of Contents
- Foreword
- Preface
- Acknowledgments
- About the Author
- Chapter 1 Introduction
- Chapter 2 Characterization of Hydrometeors
- Chapter 3 Wave Scattering by a Single Particle
- Chapter 4 Scattering and Propagation in Clouds and Precipitation
- Chapter 5 Radar Measurements and Improvement of Data Quality
- Chapter 6 Applications in Weather Observation and Quantification
- Chapter 7 Advanced Methods and Optimal Retrievals
- Chapter 8 Phased Array Radar Polarimetry
- References
- Index