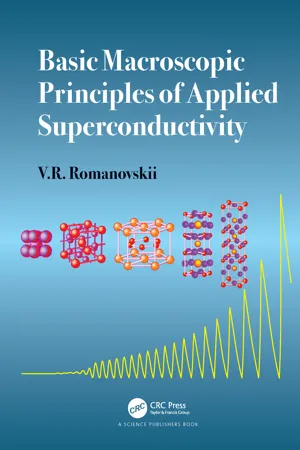
This is a test
- 392 pages
- English
- ePUB (mobile friendly)
- Available on iOS & Android
eBook - ePub
Basic Macroscopic Principles of Applied Superconductivity
Book details
Book preview
Table of contents
Citations
About This Book
This book is on applied superconductivity, which is one of the topical issues of modern science and technology. At present, the prospects for the practical application of superconductivity are obvious from both economic and practical points of view. It can reduce heat losses and reduce the weight and size of equipment, improve the reliability of electrical devices and power systems, and create new devices. As a result, the achievements of applied superconductivity make it possible to perform effective retrofitting of equipment for scientific research, in medicine, electric power, electrical engineering, transport, and to introduce new advanced technologies that provide higher efficiency, significantly reducing working costs.
Frequently asked questions
At the moment all of our mobile-responsive ePub books are available to download via the app. Most of our PDFs are also available to download and we're working on making the final remaining ones downloadable now. Learn more here.
Both plans give you full access to the library and all of Perlegoās features. The only differences are the price and subscription period: With the annual plan youāll save around 30% compared to 12 months on the monthly plan.
We are an online textbook subscription service, where you can get access to an entire online library for less than the price of a single book per month. With over 1 million books across 1000+ topics, weāve got you covered! Learn more here.
Look out for the read-aloud symbol on your next book to see if you can listen to it. The read-aloud tool reads text aloud for you, highlighting the text as it is being read. You can pause it, speed it up and slow it down. Learn more here.
Yes, you can access Basic Macroscopic Principles of Applied Superconductivity by V.R. Romanovskii in PDF and/or ePUB format, as well as other popular books in Physical Sciences & Condensed Matter. We have over one million books available in our catalogue for you to explore.
Information
1.1 SUPERCONDUCTIVITY
Superconductivity was discovered by Heike Kamerlingh Onnes in 1911 (Onnes, 1911a, 1911b, 1911c) at the University of Leiden, The Netherlands. He found that the resistance of mercury sharply disappeared below a certain temperature, which he called the critical temperature (Tc). As it was determined later, superconductivity is a new physical phase at T < Tc. This state is called the superconducting state and such materials are called superconductors. Today, the main part of pure elements having superconducting properties is known as type-I superconductors or soft superconductors. Note that the superconductors have an electrical resistivity Ļ above Tc higher than a resistivity of the good-conductivity metal, such as copper or silver in the temperature range above Tc. The superconducting state is a stable thermodynamic phase, and it is a quantum mechanical phenomenon on a macroscopic scale when the motion of all superconducting electrons is correlated with lattice vibrations. As a result, the superconducting electrons bump into nothing and create no friction. Thereby, they can transmit current with no loss of energy since the superconducting electrons pass through the complex lattice despite the fact that there are impurities and lattice inside a superconductor. Several experiments have proved the existence of permanent currents over years so that the resistance of superconducting pure metals seems practically zero, more exactly, Ļ < 10ā25 Ī©m.
Soon after the discovery of superconductivity, it was found that superconductivity may be destroyed, that is, superconductors can become normal again not only by its heating but also by the magnetic field, which calls the critical magnetic field (Bc). As a result, the field-temperature phase diagram characterizes superconductors. Onnes (Tuyn and Onnes 1926, Sizoo et al. 1926) investigated such diagrams of pure metals. According to his experiments, the following relation Bc = Bc0[1 ā (T/Tc)2] was formulated, where Bc0 is the approximation of the critical magnetic field at zero temperature. Superconductivity of pure metals exists below the dependence of Bc(T).
The superconducting state differs qualitatively from the normal (nonsuperconducting) state. Namely, besides zero electrical resistivity, the magnetic field does not penetrate a superconductor (perfect diamagnetic properties). The exclusion of magnetic fields from the bulk of a superconductor is known as the Meissner-Ochsenfeld effect (Meissner and Ochsenfeld 1933), and it is the feature of superconductors that distinguishes superconductivity from ideal conductivity. The nature of this effect cannot be explained using the macroscopic Maxwell equations because it is a quantum phenomenon. The phenomenological theory facilitates understanding of the Meissner-Ochsenfeld effect. This theory was developed by F. London and H. London (London F. and London H. 1935). They proposed the system of equations describing the microscopic electric and magnetic fields in superconductors (the so-called Londonās electrodynamics). The first equation describes the perfect electrical conductivity. The second equation leads to the solution according to which a magnetic field has an exponentially decaying form, in particular, in the 1 D approximation B = Ba exp (āx/ Ī»L), that is, the external magnetic field Ba is screened from the interior of a superconductor within a distance Ī»L, known as the London which has the values 10ā6 ā 10ā5 cm.
In 1950, Ginzburg and Landau (Ginzburg and Landau 1950) proposed the theory using thermodynamic concepts. It is based on Landauās theory of the phase transitions of the second-order to predict the superconducting electron density ns. The Ginzburg-Landau equations lead to the characteristic parameter Īŗ = Ī»L/ξ, which is equal to the ratio of the penetration depth Ī»L and the coherence length ξ;. The latter quantity may be defined as the length scale over which Ginzburg-Landauās order factor varies. The coherence length is also a measure of the length scale over which the gradual change from normal to superconducting state occurs at the external boundary of a superconductor. Therefore, it can be considered as the scale over which the superconducting electron of the density ns goes from zero at the external boundary to a constant value inside the superconductor. The Ginzburg-Landau theory is an alternative to Londonās theory. However, the Ginzburg-Landau theory does not explain the microscopic mechanisms of superconductivity. It examines the macroscopic properties of the superconductor with the aid of general thermodynamic equations. This theory is the phenomenological theory in the sense of its assumptions for describing the state transition. It is based on quantum mechanics instead of the macroscopic electromagnetic phenomena.
Bardeen, Cooper and Schrieffer advanced the understanding of superconductivity through the microscopic theory of superconductivity (Bardeen et al. 1957). It explains superconductivity at temperatures close to absolute zero. Their theory offered that atomic lattice vibrations affect the entire current in the superconductor. They force the electrons to pair up into teams that could pass all the obstacles, which cause the resistance of a normal conductor.
Abrikosov used the Ginzburg-Landau theory and investigated the properties of superconductors with Īŗ >> 1 (Abrikosov 1957). Earlier such superconducting alloys were experimentally observed by De Haas and Voogd (De Haas and Voogd 1929) and Shubnikov and his colleagues (Rjabinin and Schubnikow 1935). Abrikosov theoretically justified the existence of these materials. They were called the type-II superconductor. Later, the type-II superconductors, which may be used in practical applications, came to be called nonideal type-II superconductors or hard superconductors. Type-II superconductors are characterized by two critical fields: the first or lower(Bc1) and second or upper (Bc2) critical fields. They depend on temperature. The value of Bc2 is zero at the critical temperature of the superconductor. Type-II superconductors are in the Meissner state and exclude the external magnetic field from the bulk of the superconductor below the value of Bc1. Magnetic fields between Bc1 and Bc2 penetrate the superconductor in the form of vortexes containing the single elementary quantum ((Φ0 = 2.0678 Ā· 10ā15 Wb). The magnetic field is surrounded by a vortex supercurrent. The Cooper pair density is equal to zero at the center of the vortex meaning that the core of a vortex is a normal state. Abrikosov had shown that the normal regions organize a triangular lattice of vortexes. Such a state is called a mixed state. These states were experimentally observed in LTS and HTS (Essmann and TrƤuble 1966, Hess et al. 1991, Hug et al. 1994, Eskildsen et al. 2002). The triangular lattice is the close-packed configuration. There are large numbers of type-II superconductors. In particular, LTS and HTS are the type-II superconductors.
Since the discovery of superconductivity, many efforts were made to find superconducting materials with critical temperatures as high as possible to solve cryogenic problems. In 1973, the record value of the critical temperature reached for Nb3Ge film with a value of 23.2 K. Remarkable discovery was achieved by G. Bednorz and A. Müller (Bednorz and Müller 1986). It was shown that a copper oxide compound with the stoichiometry La2āxBaxCuO4 had the superconducting properties at about 29 K which is strongly dependent on x. The superconductor with the stoichiometry YBa2Cu3O7āx was found later. Its critical temperature is about 90 K that is higher than the boiling temperature of liquid nitrogen. Ceramics Bi2Sr2CaCu2O8+x (Bi2212) and Bi2Sr2Ca2Cu3O10+x (Bi2223), Tl2Ba2Ca2CuO8+x and HgBa2Ca2Cu3O8+x raised the upper limit of the critical temperature. The critical current of about 164 K was measured in HgBa2Ca2Cu3O8+x under the pressure of 31 GPa.
The HTS cuprates have a significant interest in applied superconductivity. However, they are highly anisotropic granular materials. Herewith, they are characterized by a weak-link effect between their grain boundaries which decreases allowable current density. They are also brittle. These features cause difficulties for applications. In spite of these handicaps, the technology of HTS fabrication makes progress. As a result, the critical currents of HTS have increased to be useful for applications. Many pilots HTS devices were manufactured: electric power cables, rotating electrical machines, generators and others. Today, HTS is used in large-scale LTS magnets as high-field inserts to produce a magnetic field higher than 22 T and are used more in the future at liquid helium temperature level, like effective current leads, as fault current limiters for electrical engineering.
Thus summarizing, it is important to note that the search for new high-temperature superconductors will undoubtedly lead to new and unexpected discoveries. Today, the scientific and techni...
Table of contents
- Cover
- Title Page
- Copyright Page
- Preface
- Introduction
- Resume
- List of Principal Symbols
- Table of Contents
- Chapter 1: Concepts and Principal Provisions of Fundamental and Applied Superconductivity
- Chapter 2: Macroscopic Electrodynamics of NonIdeal Type-II Superconductors: Isothermal Models
- Chapter 3: Physical Features of the Dissipative Phenomena in Superconductors in the Self-Field Modes
- Chapter 4: Thermo-Magnetic Phenomena in Low-Tc and High-Tc Superconductors
- Chapter 5: Thermo-Magnetic Phenomena in Composites Based on LTS and their Magnetic Instabilities
- Chapter 6: Current-Carrying Capacity of Superconducting Composites and Tapes: Formation and Destruction of Stable Current Modes
- Chapter 7: Thermal Phenomena in Composite Superconductors with an Ideal Voltage-Current Characteristic and their Thermal Stabilization Conditions
- Chapter 8: Electrophysical Features of Thermal Instabilities Occurrence in Technical Superconductors with Various Nonlinearity Types of V-I Characteristics
- References
- Index