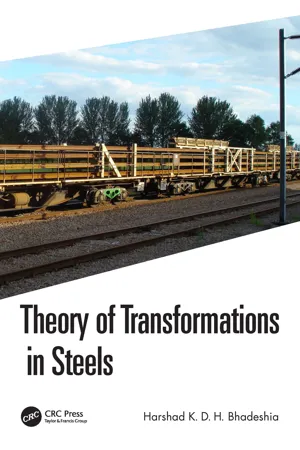
This is a test
- 574 pages
- English
- ePUB (mobile friendly)
- Available on iOS & Android
eBook - ePub
Theory of Transformations in Steels
Book details
Book preview
Table of contents
Citations
About This Book
Written by the leading authority in the field of solid-state phase transformations, Theory of Transformations in Steels is the first book to provide readers with a complete discussion of the theory of transformations in steel.
-
- Offers comprehensive treatment of solid-state transformations, covering the vast number in steels
-
- Serves as a single source for almost any aspect of the subject
-
- Features discussion of physical properties, thermodynamics, diffusion, and kinetics
-
- Covers ferrites, martensite, cementite, carbides, nitrides, substitutionally-alloyed precipitates, and pearlite
-
- Contains a thoroughly researched and comprehensive list of references as further and recommended reading
With its broad and deep coverage of the subject, this work aims at inspiring research within the field of materials science and metallurgy.
Frequently asked questions
At the moment all of our mobile-responsive ePub books are available to download via the app. Most of our PDFs are also available to download and we're working on making the final remaining ones downloadable now. Learn more here.
Both plans give you full access to the library and all of Perlegoâs features. The only differences are the price and subscription period: With the annual plan youâll save around 30% compared to 12 months on the monthly plan.
We are an online textbook subscription service, where you can get access to an entire online library for less than the price of a single book per month. With over 1 million books across 1000+ topics, weâve got you covered! Learn more here.
Look out for the read-aloud symbol on your next book to see if you can listen to it. The read-aloud tool reads text aloud for you, highlighting the text as it is being read. You can pause it, speed it up and slow it down. Learn more here.
Yes, you can access Theory of Transformations in Steels by Harshad K. D. H. Bhadeshia in PDF and/or ePUB format, as well as other popular books in Physical Sciences & Mechanics. We have over one million books available in our catalogue for you to explore.
Information
1Crystal structures and mechanisms
1.1 Allotropes of Iron
Why do metals adopt the crystal structures that they do? This no longer is a curiosity because the metallic state is so well understood that it is possible to select, from a calculation of the cohesive energies of trial structures, that which should be the most stable. Figure 1.1 shows the cohesive energy as a function of the density and crystal structure. Of all the test structures, hexagonal close-packed (hcp) iron is found to have the highest cohesion and therefore should represent the most stable form. This contradicts experience, but the calculations do not account for the ferromagnetism of body-centred cubic iron (ferrite), which would make it more stable than the hcp form. There are in fact magnetic transitions in each of the allotropes of iron, details of which are reserved for Chapter 2.
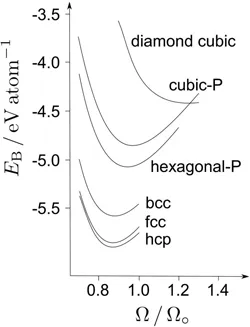
Figure 1.1 Plot of cohesive energy for 0K and 0Pa pressure versus the normalised volume per atom for a variety of crystal structures of iron. is the binding energy per atom in the crystal relative to that of a free atom. Hexagonal-P and Cubic-P are primitive structures; like the diamond cubic form, they do not exist on earth. Adapted with permission from [1]. Copyrighted by the American Physical Society.
Only three allotropes of iron occur in nature, in bulk form; Figure 1.2 shows the phase diagram for pure iron. Each point on a boundary between the phase fields represents an equilibrium state in which two phases can coexist. The triple point where the three boundaries intersect represents an equilibrium between all three co-existing phases. It is seen that in pure iron, the hexagonal close-packed form is stable only at high pressures, consistent with its high density.
There are two further allotropes which can be created in the form of thin films. Face-centred tetragonal iron can be prepared by coherently depositing iron as a thin film on a plane of a substrate such as copper with which the iron has a mismatch. The atoms in the first deposited layer replicate the positions of those on the substrate. A few monolayers can be forced into coherency in the plane of the substrate with a corresponding distortion normal to the substrate. This gives the deposit a face-centred tetragonal structure which in the absence of any mismatch would be face-centred cubic [2, 3]. Eventually, as the film thickens during the deposition process to beyond about ten monolayers (on copper), the structure relaxes to the low-energy bcc form, a process accompanied by the formation of dislocation defects which accommodate the misfit with the substrate.
Growing iron on a misfitting surface of a face-centred cubic substrate similarly causes a distortion along the surface normal, giving trigonal iron [4].1 Graphene is a single layer of carbon atoms that has a hexagonal structure with a lattice parameter of 0.245nm. The crystal is seldom perfect, often containing holes. Compounds used in its manufacture provide a source of iron atoms that can attach themselves to the edges of these holes, building up into monoatomic layers that are suspended in the graphene films. These âfree-standingâ single-atom-thick two-dimensional arrays of iron form a square lattice with a parameter 0.265 nm [5]. This particular structure has been suggested to be a consequence of lattice matching with the âarmchairâ configuration of carbon atoms at the graphene edges where the bonding between the iron and carbon atoms is stronger than when the iron attaches to the âzig-zagâ edges of the graphene [5]. The largest stable monolayer of iron on holes in graphene is found to be about 3nm2 in area.
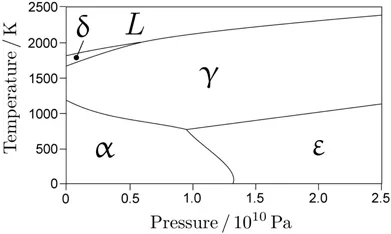
Figure 1.2 Temperature versus pressure equilibrium phase diagram for pure iron, based on assessed thermodynamic data. The triple point temperature and pressure are 490âC and 11GPa respectively. , and refer to ferrite, austenite and -iron respectively. ÎŽ is simply the higher temperature designation of . Diagram courtesy of Shaumik Lenka, calculated using the database âTCFE8â, and ThermoCalc version 2015b.
Table 1.1 lists some transformation temperatures and thermodynamic data for the natural allotropes of iron. The free energy changes during the solid-state transformations are really quite small, even when compared with the energy associated with the magnetic disordering of ferrite. In a way, this is a reflection of the fact that their crystal structures are not all that different (Figure 1.3). For example, assuming a hard-sphere model, the crystal structure of austenite and -iron can be generated by stacking close-packed layers of iron atoms in such a way that each successive layer sits in the depressions of the underlying layer. There are in each layer, two types of depressions, so the close-packed layers can be stacked either in the sequence or . The former sequence, which has a stacking period of three, generates the austenite structure, whereas the latter gives the hcp structure of -iron with a stacking period of two. The best comparison of the relative densities of the phases is made at the triple point where the allotropes are in...
Table of contents
- Cover
- Half Title
- Title
- Copyright
- Dedication
- Contents
- Preface
- Author
- Acronyms etc.
- Nomenclature
- Chapter 1 Crystal structures and mechanisms
- Chapter 2 Thermodynamics
- Chapter 3 Diffusion
- Chapter 4 Ferrite by reconstructive transformation
- Chapter 5 Martensite
- Chapter 6 Bainite
- Chapter 7 WidmanstÀtten ferrite
- Chapter 8 Cementite
- Chapter 9 Other Fe-C carbides
- Chapter 10 Nitrides
- Chapter 11 Substitutionally alloyed precipitates
- Chapter 12 Pearlite
- Chapter 13 Aspects of kinetic theory
- Author index
- Subject index