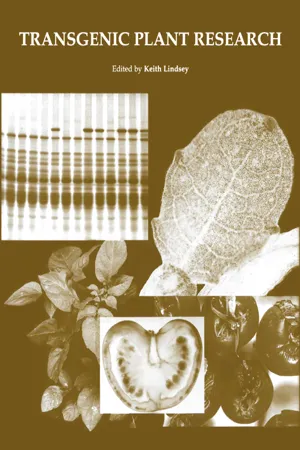
This is a test
- 304 pages
- English
- ePUB (mobile friendly)
- Available on iOS & Android
eBook - ePub
Transgenic Plant Research
Book details
Book preview
Table of contents
Citations
About This Book
This text is split into four main sections: gene transfer techniques; transgenic approaches to gene isolation; manipulation of plant development, biochemistry and physiology; and predictability of transgene expression.
Frequently asked questions
At the moment all of our mobile-responsive ePub books are available to download via the app. Most of our PDFs are also available to download and we're working on making the final remaining ones downloadable now. Learn more here.
Both plans give you full access to the library and all of Perlegoâs features. The only differences are the price and subscription period: With the annual plan youâll save around 30% compared to 12 months on the monthly plan.
We are an online textbook subscription service, where you can get access to an entire online library for less than the price of a single book per month. With over 1 million books across 1000+ topics, weâve got you covered! Learn more here.
Look out for the read-aloud symbol on your next book to see if you can listen to it. The read-aloud tool reads text aloud for you, highlighting the text as it is being read. You can pause it, speed it up and slow it down. Learn more here.
Yes, you can access Transgenic Plant Research by Alan R. Lindsey in PDF and/or ePUB format, as well as other popular books in Biological Sciences & Botany. We have over one million books available in our catalogue for you to explore.
Information
Agrobacterium-mediated Plant Transformation: a Scientifically Intriguing Story with Significant Applications | 1 |
1 Laboratorium voor Genetica, Department of Genetics, Flanders Interuniversity Institute for Biotechnology, Universiteit Gent, K.L. Ledeganckstraat 35, B-9000 Gent, Belgium; e-mail: [email protected]
2 Vakgroep Plantaardige Productie, Faculteit Landbouwkundige en Toegepaste Biologische Wetenschappen, Universiteit Gent, B-9000 Gent, Belgium
summary
The most widely used method for the introduction of new genes into plants is based on the natural DNA transfer capacity of Agrobacterium tumefaciens. Over the past decades, intensive studies on the mechanism of T-DNA transfer have resulted in a reasonably detailed insight into this process. This research revealed that the genetic transformation of plant cells by Agrobacterium evolved by adaptation of processes that existed long before the evolution of the plantâAgrobacterium association. This conclusion holds true for the two-component signal transduction system leading to induction of the virulence genes and for the mechanism responsible for T-DNA transfer to plant cells, which is very similar to that of bacterial conjugation. Integration of the T-DNA in the plant chromosomes is accomplished by the plantâs illegitimate recombination system, but with the help of agrobacterial proteins, which enhance the precision and the efficiency of the integration process. This chapter starts with an overview of the current knowledge on the molecular basis of Agrobacterium-mediated plant transformation. The main emphasis is on the role of the virulence proteins and on recent insights into the later steps of the transformation process. Then, a summary is given on the characteristics of the T-DNA inserted into the plant nuclear DNA. The chapter concludes with a critical assessment of the different approaches that have been used to exploit Agrobacterium tumefaciens, a natural âengineerâ, for the production of transgenic plants.
Key words: Agrobacterium tumefaciens, plant transformation, regeneration, selectable marker, T-DNA, virulence.
Introduction
The dawn of plant molecular biology and genetic engineering was basically the consequence of the discovery and study of the plant pathogen Agrobacterium tumefaciens. In nature, this Gram-negative soil bacterium causes tumour formation (so-called crown galls) on a large number of dicotyledonous as well as some monocotyledonous plant species and Gymnosperms (De Cleene and De Ley, 1976). These tumours can be grown in vitro in simple media without the continued presence of the bacterium (Braun and White, 1943). Although A. tumefaciens had been studied for nearly 100 years, it was the advent of recombinant DNA technology that led to the analysis of the biological principles underlying the Agrobacteriumâplant cell interaction and to the modification of the system for plant genetic engineering. The crown gall disease has been shown to be due to the transfer of a specific DNA fragment, the T-DNA (transferred DNA), from a large tumour-inducing (Ti) plasmid within the bacterium (Zaenen et al., 1974) to the plant cell (summarized in Figure 1.1). After transfer, the T-DNA becomes integrated into the plant nuclear genome (Chilton et al., 1977; Schell et al., 1979) and its subsequent expression leads to the crown gall phenotype. Some of the T-DNA genes are responsible for the synthesis or modification of basic plant growth factors, such as auxins and cytokinins. The higher hormone levels produced in a way that escapes the plant cell control alters the normal differentiation of plant cells and provokes neoplastic growth.
Other T-DNA genes direct the synthesis of particular amino acid or sugar derivatives that are not normally found in plant cells. One of these is octopine, a condensation product of arginine with pyruvic acid that was formerly only known from Octopus, hence its name. These plant tumour-specific compounds are now generally referred to as opines (Ménagé and Morel, 1965; Ellis and Murphy, 1981; for a review, see Dessaux et al., 1992). Based on the kind of opines encoded by the corresponding Ti plasmids, broad host range agrobacteria can be classified as octopine, nopaline, agropine, succinamopine or chrysopine strains (Dessaux et al., 1992; Vaudequin-Dransart et al., 1995). The opines formed in the tumours can be metabolized by the agrobacteria that incited the tumour, but not by most other soil microbes (Tempé and Petit, 1982). In this way, Agrobacterium creates a favourable niche for itself by genetic modification of plant cells.
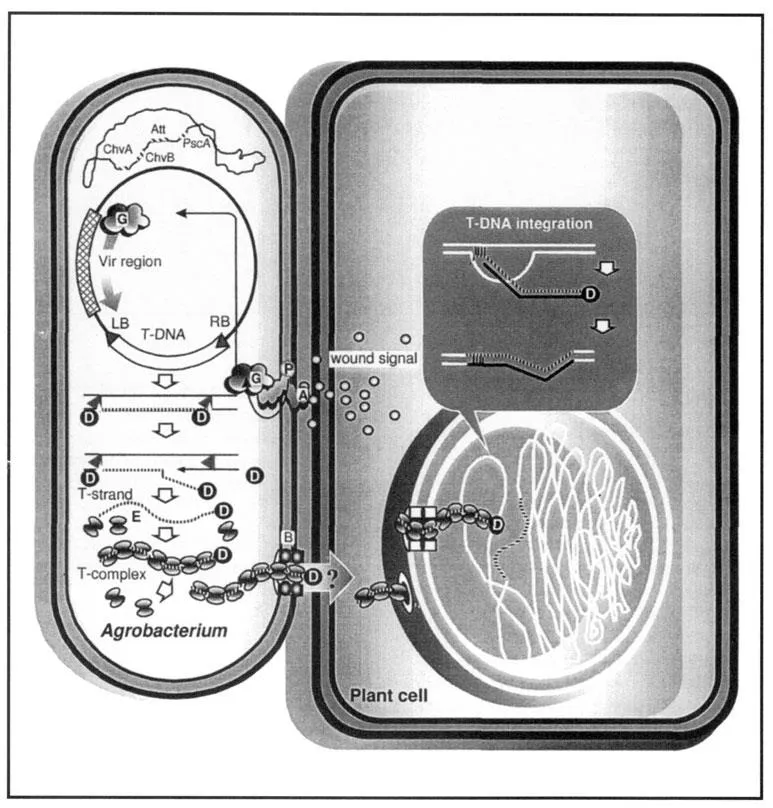
Three bacterial genetic elements are required for T-DNA transfer to plants. The first of these elements are the T-DNA border sequences that consist of 24-or 25-bp direct repeats flanking and defining the T-DNA (Van Haaren et al., 1988). Usually, all DNA sequences between the borders are transferred to the plant. The borders are the only sequences required in cis for T-DNA transfer (Zambryski et al., 1983). The second element consists of the virulence (vir) genes encoded by the Ti plasmid in a region outside of the T-DNA (for a review, see Hooykaas and Beijersbergen, 1994). VirA and VirG form a two-component regulatory system responsible for transcriptional activation of the vir operons (Stachel and Zambryski, 1986a). The other vir genes are involved in processing of the T-DNA from the Ti plasmid and in T-DNA transfer from the bacterium to the plant cell. Certain Vir proteins promote T-DNA targeting to the nucleus and are probably involved in precise T-DNA integration into the plant DNA. The third bacterial element necessary for T-DNA transfer consists of a number of chromosomal genes, of which some are important for attachment of the bacterium to the plant cell.
A. rhizogenes causes the plant disease known as hairy root, characterized by the fast proliferation of roots at the wound site infected by the bacterium. Although A. rhizogenes Ri plasmids show only limited homology with Ti plasmids (e.g., White and Nester, 1980; Risuleo et al., 1982; Huffman et al., 1984), the basic mechanisms of infection and gene transfer are very similar (Tepfer, 1989; Weising and Kahl, 1996).
The Role of the Bacterial Virulence Genes in the Infection Process
Plant Signal Molecules Attract the Agrobacteria and Activate the T-DNA Transfer Process
Chemotaxis plays an important role in the recognition of susceptible (wounded) plants by Agrobacterium (Hawes and Smith, 1989). As for many other bacteria, Agrobacterium is chemotactic to a wide range of sugars and amino acid compounds, but it can also recognize specific secondary plant metabolites, such as phenolics produced by damaged plant cells. Several reports from one research group (Ashby et al., 1987, 1988; Shaw et al., 1988) demonstrate that acetosyringone and related compounds elicit a Ti plasmid-dependent chemotaxis of Agrobacterium. This observation could, however, not be reproduced by other groups using a different Agrobacterium strain (Parke et al., 1987; Hawes and Smith, 1989), which indicates that the released sugars might be the more general chemotactic signal for the agrobacteria.
A second early step in infection is the binding of bacteria to target plant cells. Potentially, plant vitronectin-like proteins may represent receptors responsible for this specific interaction (Wagner and Matthysse, 1992). The initially loose binding is then stabilized by the bacterial synthesis of cellulose fibrils. A number of bacterial mutants affected in attachment have been isolated. All genes involved in attachment are located on the Agrobacterium chromosome and mutants in these loci (chvA, chvB, pscA or exoC, and att) are avirulent on many plant species (Douglas et al., 1985; Matthysse, 1987; Thomashow et al., 1987). chvA, chvB, and pscA are involved in the synthesis and export of cyclic ÎČ-1,2-glucan (Cangelosi et al., 1987, 1989; Zorreguieta et al., 1988; Uttaro et al., 1990). The lack of this ÎČ-1,2-glucan results in various changes in the cell envelope, leading not only to attachment deficiency, but also to reduced motility (Douglas et al., 1982). Interestingly, pscA mutants efficiently transfer T-DNA to the plant nucleus when the bacterial cells are micro-injected into the cytoplasm of plant cells (Escudero et al., 1995). A more direct role in attachment has been demonstrated for rhicadhesin, a calcium-binding protein located on the bacterial cell surface (Smit et al., 1989; Swart et al., 1993, 1994). Indeed, chvB mutants can be rescued by the addition of active rhicadhesin. Several att mutants have been mapped to one chromosomal DNA region, from which a 10-kb region has recently been sequenced (Matthysse et al., 1996). In this way, open reading frames (ORFs) were found with similarity to ATP-binding cassette (ABC) transport systems possibly involved in the secretion of substances required for bacterial attachment. Besides these bacterial mutants, which have been useful for identifying the different chromosomal genes involved in attachment, recent studies have revealed a natural variation in Agrobacterium-binding efficiencies of different Arabidopsis thaliana ecotypes. In several of these ecotypes, recalcitrance to tumorigenesis results from decreased adhesion of Agrobacterium to inoculated root explants (Nam et al., 1997).
Whereas the chv genes are constitutively expressed, the Ti plasmid-encoded vir genes are...
Table of contents
- Cover
- Half Title
- Title Page
- Copyright Page
- Table of Contents
- Preface
- Contributors
- Part 1: Gene Transfer Techniques
- Part 2: Transgenic Approaches to Gene Isolation
- Part 3: Manipulation of Plant Development, Biochemistry and Physiology
- Part 4: Predictability of Transgene Expression
- Index