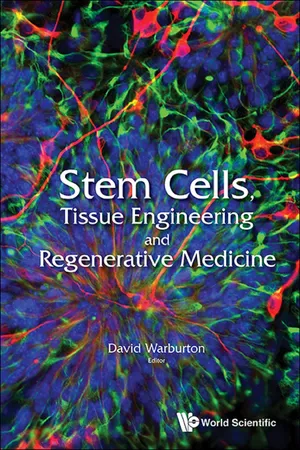
- 552 pages
- English
- ePUB (mobile friendly)
- Available on iOS & Android
Stem Cells, Tissue Engineering And Regenerative Medicine
About This Book
Stem cells, tissue engineering and regenerative medicine are fast moving fields with vastly transformative implications for the future of health care and capital markets. This book will show the state of the art in the translational fields of stem cell biology, tissue engineering and regenerative medicine. The state of developments in specific organ systems, where novel solutions to organ failure are badly needed such as the lungs, kidney and so forth, are discussed in various chapters. These present and future advances are placed in the context of the overall field, offering a comprehensive and quick up-to-date drink from the fountain of knowledge in this rapidly emerging field.
This book provides an investigator-level overview of the current field accessible to the educated scientific generalist as well as a college educated readership, undergraduates and science writers, educators and professionals of all kinds.
Contents:
- Developmental Biology, Regenerative Medicine and Stem Cells: The Hope Machine is Justified (David Warburton)
- Towards Broader Approaches to Stem Cell Signaling and Therapeutics (Edwin Jesudason)
- Pluripotent Stem Cells from the Early Embryo (Claire E Cuddy and Martin F Pera)
- The First Cell Fate Decision During Mammalian Development (Melanie D White and Nicolas Plachta)
- Asymmetric Cell Divisions of Stem/Progenitor Cells (Ahmed HK El-Hashash)
- Microenvironmental Modulation of Stem Cell Differentiation with Focus on the Lung (Shimon Lecht, Collin T Stabler, Seda Karamil, Athanasios Mantalaris, Ali Samadikuchaksaraei, Julia M Polak and Peter I Lelkes)
- Smart Matrices for Distal Lung Tissue Engineering (Mark J Mondrinos and Peter I Lelkes)
- Skin Stem Cells and Their Roles in Skin Regeneration and Disorders (Chao-Kai Hsu, Chao-Chun Yang and Shyh-Jou Shieh)
- Stem Cell Recruitment and Impact in Skin Repair and Regeneration (Tim Hsu, Tai-Lan Tuan and Yun-Shain Lee)
- Epigenetic and Environmental Regulation of Skin Appendage Regeneration (Ting-Xin Jiang, Chih-Chiang Chen, Michael W Hughes, Cheng-Ming Chuong and Randall Widelitz)
- Cranial Neural Crest: An Extraordinarily Migratory and Multipotent Embryonic Cell Population (Samuel G Cox and J Gage Crump)
- Modeling Neurodegenerative Diseases and Neurodevelopmental Disorders with Reprogrammed Cells (Kate E Galloway and Justin K Ichida)
- Cytokine Regulation of Intestinal Stem Cells (Philip E Dubé, Unice J K Soh and D Brent Polk)
- The Intestinal Stem Cell Niche and Its Regulation by ErbB Growth Factor Receptors (Dana Almohazey and Mark R Frey)
- Tissue Engineering: Intestine (Avafia Y Dossa, Kathy A Schall, Tracy C Grikscheit and Christopher P Gayer)
- Liver Stem and Progenitor Cells in Development, Disease and Regenerative Medicine (Nirmala Mavila and Kasper S Wang)
- Lung Mesenchymal Stem Cells (Wei Shi)
- FGF Signaling in Lung Stem and Progenitor Cells (Soula Danopoulos and Denise Al Alam)
- Bioengineering Distal Airways (Christine Finck and Todd Jensen)
- The Isolation and Molecular Characterization of Cancer Stem Cells (Aggressive Endophenotypes) in Individual Lung Cancers (Raj K Batra, Scott Oh and Saroj Basak)
- Mesenchymal Stromal Cell-Based Therapies for Lung Diseases and Critical Illnesses (Fernanda Cruz, Patricia RM Rocco and Daniel J Weiss)
- Heart Regeneration and Repair: What We Have Learned from Model Organisms (Laurent Gamba, Michael R Harrison and Ching-Ling Lien)
- Leveraging Structure-Based Rational Drug Design and Nanotechnology to Destroy Leukemic Stem Cells (Fatih M Uckun, Jianjun Cheng, Cheney Mao and Sanjive Qazi)
- Placenta-Derived Stem Cells: Development and Preclinical Applications for Regenerative Medicine (Jennifer Izumi Divine, Hee Kyung Jung and Toshio Miki)
- Stem Cells in the Real World: Environmental Impacts (Theresa M Bastain, Lu Gao and Frank D Gilliland)
- Establishing a Research Grade Human Pluripotent Stem Cell Laboratory (Laura-Marie Nucho and Victoria Fox)
Readership: Stem cell and tissue engineering scientists, patient advocates, educated laypeople, high school science students, undergraduate students, graduate students, physicians and surgeons.
Key Features:
- This book presents up-to-date latest breakthroughs and near future applications
- Bench to bedside
- This book features potential cures
Frequently asked questions
Information
1
Pluripotent Stem Cells from the Early Embryo
Claire E. Cuddyā and Martin F. Pera*ā ,ā”,§
ā University of Melbourne
ā”Florey Neuroscience and Mental Health Institute
§Walter and Eliza Hall Institute of Medical Research
Introduction
Pluripotent Stem Cells have Unique Properties
Mouse Embryonic Development Provides the Conceptual Basis for Understanding PSC
Normal Pluripotent Stem Cells Were First Derived From the Epiblast
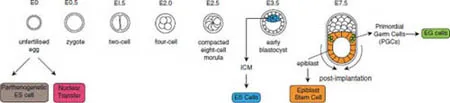
Pluripotent Stem Cells can be Generated From Different Stages of Development and by Cellular Reprogramming
Type of Pluripotent... |
Table of contents
- Cover
- Halftitle
- Title Page
- Copyright Page
- Contents
- Introduction: Developmental Biology, Regenerative Medicine and Stem Cells: The Hope Machine is Justified
- Foreword: Towards Broader Approaches to Stem Cell Signaling and Therapeutics
- Chapter 1 Pluripotent Stem Cells from the Early Embryo
- Chapter 2 The First Cell Fate Decision During Mammalian Development
- Chapter 3 Asymmetric Cell Divisions of Stem/Progenitor Cells
- Chapter 4 Microenvironmental Modulation of Stem Cell Differentiation with Focus on the Lung
- Chapter 5 Smart Matrices for Distal Lung Tissue Engineering
- Chapter 6 Skin Stem Cells and Their Roles in Skin Regeneration and Disorders
- Chapter 7 Stem Cell Recruitment and Impact in Skin Repair and Regeneration
- Chapter 8 Epigenetic and Environmental Regulation of Skin Appendage Regeneration
- Chapter 9 Cranial Neural Crest: An Extraordinarily Migratory and Multipotent Embryonic Cell Population
- Chapter 10 Modeling Neurodegenerative Diseases and Neurodevelopmental Disorders with Reprogrammed Cells
- Chapter 11 Cytokine Regulation of Intestinal Stem Cells
- Chapter 12 The Intestinal Stem Cell Niche and Its Regulation by ErbB Growth Factor Receptors
- Chapter 13 Tissue Engineering: Intestine
- Chapter 14 Liver Stem and Progenitor Cells in Development, Disease and Regenerative Medicine
- Chapter 15 Lung Mesenchymal Stem Cells
- Chapter 16 FGF Signaling in Lung Stem and Progenitor Cells
- Chapter 17 Bioengineering Distal Airways
- Chapter 18 The Isolation and Molecular Characterization of Cancer Stem Cells (Aggressive Endophenotypes) in Individual Lung Cancers
- Chapter 19 Mesenchymal Stromal Cell-Based Therapies for Lung Diseases and Critical Illnesses
- Chapter 20 Heart Regeneration and Repair: What We Have Learned From Model Organisms
- Chapter 21 Leveraging Structure-Based Rational Drug Design and Nanotechnology to Destroy Leukemic Stem Cells
- Chapter 22 Placenta-Derived Stem Cells: Development and Preclinical Applications for Regenerative Medicine
- Chapter 23 Stem Cells in the Real World: Environmental Impacts
- Chapter 24 Establishing a Research Grade Human Pluripotent Stem Cell Laboratory
- Index