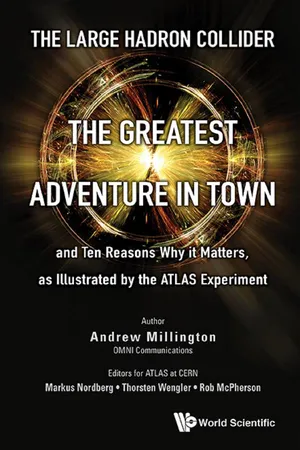
eBook - ePub
The Large Hadron Collider
The Greatest Adventure in Town and Ten Reasons Why it Matters, as Illustrated by the ATLAS Experiment
This is a test
- 292 pages
- English
- ePUB (mobile friendly)
- Available on iOS & Android
eBook - ePub
The Large Hadron Collider
The Greatest Adventure in Town and Ten Reasons Why it Matters, as Illustrated by the ATLAS Experiment
Book details
Book preview
Table of contents
Citations
About This Book
When the discovery of the Higgs Boson at CERN hit the headlines in 2012, the world was stunned by this achievement of modern science. Less well appreciated, however, were the many ways in which this benefited wider society.
The Large Hadron Co
Frequently asked questions
At the moment all of our mobile-responsive ePub books are available to download via the app. Most of our PDFs are also available to download and we're working on making the final remaining ones downloadable now. Learn more here.
Both plans give you full access to the library and all of Perlegoās features. The only differences are the price and subscription period: With the annual plan youāll save around 30% compared to 12 months on the monthly plan.
We are an online textbook subscription service, where you can get access to an entire online library for less than the price of a single book per month. With over 1 million books across 1000+ topics, weāve got you covered! Learn more here.
Look out for the read-aloud symbol on your next book to see if you can listen to it. The read-aloud tool reads text aloud for you, highlighting the text as it is being read. You can pause it, speed it up and slow it down. Learn more here.
Yes, you can access The Large Hadron Collider by Andrew J Millington, Markus Nordberg;Thorsten Wengler;Rob McPherson; in PDF and/or ePUB format, as well as other popular books in Scienze fisiche & Fisica nucleare. We have over one million books available in our catalogue for you to explore.
Information
Topic
Scienze fisicheSubtopic
Fisica nucleareChapter 1
The Physics Itself and Why it Needed the LHC
There are some days you never forget. Not because they are unique personal experiences but because they seem to represent something special about the human spirit, and often something that unifies us as human beings. Those old enough to remember the first image of the full Earth taken from space in the early days of lunar exploration (or have seen film of that mission) will surely recall a rare frisson of excitement at what was clearly a historic moment ā a moment for all humankind. It seemed to be a statement of our common occupancy of our planet, linked to a shared urge for exploration.
For those who visit the Large Hadron Collider (the LHC) at CERN near Geneva for the first time, and take the lift down the pit of the giant ATLAS experiment, the occasion will surely also be seared on the memory. It is not only the scale of the machine, the 27 kilometre accelerator and the detector the size of a six-storey building. One's awe is overlaid by the knowledge that the peoples of the world have come together to explore the deepest secrets of nature, something quite inspiring and stunning in itself. And if one is privileged to be part of a guided tour, one soon realises that beneath that philosophical perspective lie layers of revelation on what scientists and engineers have achieved in, or as a result of, the decades of experimental physics at CERN. Perhaps, the most famous is the creation of the World Wide Web, not visible of course on your first ā or any ā visit but a direct consequence of the scale and fundamental nature of the enterprise called CERN.
In fact there are many rich veins of achievement from CERN research. So the first great success of the new era, the discovery of the so-called Higgs boson, offers a timely milestone for us to look afresh at everything that now makes up this unique operation, and its consequences. The ATLAS experiment (ATLAS stands for A ToroidaL ApparatuS (for LHC)), is centred on the enormous ATLAS detector which straddles the particle accelerator, the LHC. It is one of the two large experiments at CERN which confirmed in 2012 the existence of the heralded Higgs boson. We shall focus on the ATLAS experiment to reveal some of the amazing outcomes from the collective effort on ATLAS from 38 countries across the world. These embrace the areas of physics, technology, IT, sociology, philosophy, politics, economics, industry, developments in medicine and more.
Many of the practical benefits of particle physics over the years are well known to us all, but their origins are often not recognised. The touch screen familiar in so many devices today was invented at CERN. Such so-called spin-offs include the World Wide Web ā an extraordinary collateral benefit that has re-shaped our world. The consequences of a modern particle physics experiment now touch many areas of life, as the chapter headings in this book indicate. Whereas all big science projects have consequences beyond their own domain, we shall see that there are some clear reasons why the accelerators and experiments at CERN have a special impact.
The driver of everything is the ageless quest for understanding at a fundamental level of what are the key constituents of matter, into what intellectual framework do they fit, and how can we detect them beyond reasonable doubt. So, in this first chapter weāll explore why the physics led CERN to build the Large Hadron Collider, how the engineering, cost and politics came together to make it happen, and how the ATLAS experiment sought to pin down the elusive particles predicted to appear at the new energies achievable at the LHC.
How the ATLAS Experiment Happened
The constraints on achieving a viable accelerator and detector are huge. The scale of the Large Hadron Collider itself is governed by the need to speed up the proton beams to very close to the speed of light. Being charged particles (a proton is the positively charged nucleus of the hydrogen atom) they can be accelerated by electromagnetic fields, but the faster they go the harder the beam is to bend. Charged particles give off electromagnetic radiation when their paths are changed, so the accelerator ring has to be as gentle a bend as possible to limit this and the corresponding force needed to keep the beam in circuit. It becomes a trade-off between accelerator size and the strength of bending force required. But this circular geometry allows identical beams to be sent in opposite directions around the ring.
The beams can then be made to collide at specific points chosen for the detectors. Similar arguments of scale apply to the detectors. A detector like ATLAS has to measure the energies and trajectories of particles created as the proton beams or bunches collide. These resulting particles also travel at high speeds so powerful magnets are needed again to bend their paths and help identify their energies and origins. So how did the physicists identify the critical size and parameters for the LHC and its experiments? How did the demands of the physics shoe in with the technological constraints and the cost?
In this book, we donāt intend to go in depth into the physics. There are many papers and publications which do that. What we aim to do is to give an overview of the physics to aid an appreciation of the whole enterprise. This includes the cultural impact of the physics on society at large. Our intention here is to show the many faceted nature of the ATLAS experiment in the context of the wider CERN and particle physics world. For those who contend that the search for the Higgs boson and other new particles may be exciting, but itās expensive when there are many more pressing practical problems, we will show that the upfront cost is a poor guide to value as so much else flows from the ATLAS/CERN endeavour. But the gains from building the 27 kilometre accelerator and its big detectors have not been widely trumpeted, and certainly not as a coherent presentation. So thatās where weāre coming from.
Because so many key insights are well expressed by both the top brains and personalities within ATLAS and from non-physics observers alike, we shall draw on their wisdom in covering our designated agenda. This approach stems from the many revealing interviews carried out while preparing films for ATLAS and CERN, but is extended to involve further perspectives, for example, from industrial companies who collaborated with ATLAS and from different ATLAS physicists.
So to summarise, there are essentially four elements in creating a successful particle experiment at new energies:
Having clear aims for the physics, in other words knowing what you expect to find and what might emerge in unchartered waters
Having engineering strategies to realise both the particle accelerator and the detector/experiment ā the two hardware-based components for the project
Securing sufficient finance and support by understanding the interests of different people and organisations
Having or creating an organisational structure that will deliver all aspects of the project
All these factors become more fascinating the more one probes. And they will permeate the diverse themes addressed here in different chapters. So what was the central rationale for creating the Large Hadron Collider and the ATLAS experiment? The other big experiment called CMS (CMS stands for Compact Muon Solenoid) of course shares some of the attributes of ATLAS but differs in a variety of ways, and from time to time contributors and ourselves will allude to CMS on specific issues. But, ATLAS is our focus.
By 2014, there were universities, institutes and laboratories from 38 countries involved in the ATLAS experiment, with around 3,000 physicists participating. The discovery of the Higgs particle, or Higgs boson as it is called, set the world alight not only within science. But in physics, it made being part of one of the two big experiments that found the Higgs even more cherished. It was the fact that the hunt for the Higgs was so demanding and also of fundamental relevance that gave it its appeal. The Higgs boson ā like some other elementary particles ā lives for only a tiny fraction of a second, and isnāt seen directly. It is identified by the way it is expected to decay into other particles, and by its expected energy. So the task for the experimenters is akin to that of a really high-tech detective, looking not for the suspect but for fingerprints or shoe-indents that might betray his or her existence. The detector itself has been described as a supermicroscope linked to an ultra-high-speed digital camera.
The inputs are not light but high speed protons. These come from the counter-rotating beams inside the Large Hadron Collider (protons are hydrogen ions and are the lightest of the type of particle called hadrons. The ions of heavier elements are also hadrons, and can also be made to circulate in the LHC). These protons are made to collide inside the four experiments at the LHC. The currency which the physicists use to measure the energy of all the particles is electronvolts, or eV. And GeV are Giga electronvolts or a thousand million electronvolts.
The scale of everything at the LHC and ATLAS is stunning. And the style of managing the experiments is unique and intriguing. What is clear is that it works, and we shall explore why in a later chapter. The leader of ATLAS is called a Spokesperson, and there have been three occupants of this post since ATLAS was formed from the amalgamation of two designs in the 1990s. Weāll pick up some of their key insights throughout the book.
Peter Jenni, the first Spokesperson, was the early inspiration for ATLAS. With a career at the cutting edge of CERN research going back to one of the two experiments which discovered the W and Z particles in the 1980s, his ability to steer a growing number of gifted and opinionated physicists towards an agreed design for ATLAS is widely credited as central to its success. In Peter Jenniās words, the journey was quite precarious, requiring strategies that were practical as well as inspiring.
āItās of course a question of all the risk assessments, of assessing whether you can do it in time. It was clear what we wanted, even at the beginning of ATLAS, but itās important to put in the time line, how long can you do R&D (the Research and Development of detector elements before a design is settled). So we had to fix very early the choice of magnet, as a toroid magnet, even before ATLAS was fully approved.ā
The shape of the magnet, a toroid like a doughnut, was a seminal design feature of ATLAS. It was deemed to provide the best shape of magnetic field to bend the paths of the muons produced as a result of the proton collisions, and give optimal information about them. What was essential in such a project was to fix some of the parameters early on to avoid having an uncontrollable sea of options which would be hard to make converge into an agreed design.
By the time of the discovery of the Higgs particle in 2012, Fabiola Gianotti had been at the helm of the ATLAS experiment for four years, the second Spokesperson for this growing experiment. Like Peter Jenni before her, she revelled in the completely international nature of the research effort, which was seen as a natural level for the ATLAS project both intellectually and in terms of resources.
āWhat is nice in this work (as Spokesperson) is that you have to face a variety of different aspects of the experiment every day, and this stimulates and opens your mind very much. In addition, dealing with people from all over the world in such a big collaboration is also a very enriching experience.ā
Following the discovery of the Higgs boson, the next phase of ATLAS is being led by a new Spokesperson, Dave Charlton. As with all senior posts within ATLAS, he was elected by the Institutions participating in ATLAS (consisting of institutes such as national laboratories or university departments) for a fixed term, and not appointed. Dave Charlton is taking a ship of proven strength into quite new waters.
āWhen we start again (in 2015), we will have this higher energy, high luminosity or beam intensity, the rates we are producing higher mass particles will be much higher. So if there are new things there, then we should see them much quicker than we would have done if we would have stayed at the current 8TeV collision energy (a TeV is a million, million electron volts, much larger than in any previous particle accelerator), and in fact if they are very heavy, we would not have seen them at 8TeV.ā
A Guided Tour of the Physics
So what is the physics challenge? Why is the Higgs particle so important, and what else might appear within the decades of operation of the ATLAS experiment? It should be remembered that we are looking at conditions in the cauldron that was the early universe, where conditions were very different from those on Earth today. That is why it needs a high energy particle accelerator to re-create them, and why physicists wrestle with concepts that are quite removed from our everyday world. But there is a cunning logic which links them to things we know, like mass and energy, which themselves are connected via Einsteinās famous equation E = mc2. It is this logic which allows those with little knowledge of physics an entrĆ©e to this exotic world.
A leading theoretical physicist within the ATLAS collaboration, Ian Hinchliffe, is based at UC Berkeley in California. He looks first at an enduring puzzle in physics, why different particles have the mass they do.
āThe ATLAS experiment when it was designed (in the early nineteen-nineties) was aimed at finding the origin of mass. Why is the proton so much heavier than the electron? Why is the Z that was studied so much here at CERN, at the Large Electron Positron collider (the previous accelerator at CERN called LEP), why is that larger than the proton mass? And in the standard model of high energy physics, one single particle called the Higgs boson is responsible for the mass of the electron and the Z (or as the physicists would normally put it: elementary particles acquire mass because of their interaction with the BroutāEnglertāHiggs field. The Higgs boson is a...
Table of contents
- Cover
- Halftitle
- Title
- Copyright
- Preface
- About the Author
- Contents
- Chapter 1 The Physics Itself and Why it Needed the LHC
- Chapter 2 The Philosophical Context, Drawing on Content Presented by Professor Renn
- Chapter 3 The ATLAS Detector as an Endeavour
- Chapter 4 The World Comes Together ā Spreading Expertise and Garnering Resources
- Chapter 5 The IT āMiracleā of the LHC/ATLAS Grid, Following on the Success of the WEB
- Chapter 6 The Sociology of ATLAS and CERN; Models for a Future World
- Chapter 7 The Economic Take on ATLAS: The Options Approach and Industrial Examples
- Chapter 8 Particle Physics Transforms Medicine: Latest Examples from ATLAS and CERN
- Chapter 9 ATLAS and CERN as an Inspiration for Research and Recruitment Across Science and Technology
- Chapter 10 Further Implications of the Physics, and Next Steps, Including Extra Dimensions of Space, Supersymmetry and Dark Matter
- Glossary