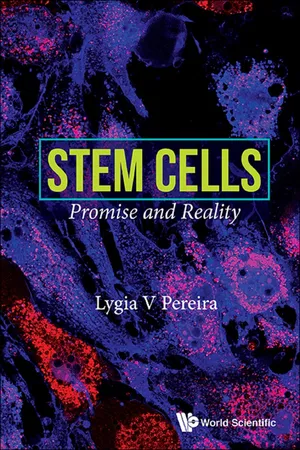
This is a test
- 108 pages
- English
- ePUB (mobile friendly)
- Available on iOS & Android
eBook - ePub
Book details
Book preview
Table of contents
Citations
About This Book
Stem Cells: Promises and Reality will tell you everything you have always wanted to know about stem cells, but could not understand the field from elsewhere.
Stem cells are the great therapeutic promise of the century, and this evolving fi
Frequently asked questions
At the moment all of our mobile-responsive ePub books are available to download via the app. Most of our PDFs are also available to download and we're working on making the final remaining ones downloadable now. Learn more here.
Both plans give you full access to the library and all of Perlego’s features. The only differences are the price and subscription period: With the annual plan you’ll save around 30% compared to 12 months on the monthly plan.
We are an online textbook subscription service, where you can get access to an entire online library for less than the price of a single book per month. With over 1 million books across 1000+ topics, we’ve got you covered! Learn more here.
Look out for the read-aloud symbol on your next book to see if you can listen to it. The read-aloud tool reads text aloud for you, highlighting the text as it is being read. You can pause it, speed it up and slow it down. Learn more here.
Yes, you can access Stem Cells by Lygia V Pereira in PDF and/or ePUB format, as well as other popular books in Medicine & Gynecology, Obstetrics & Midwifery. We have over one million books available in our catalogue for you to explore.
Information
Topic
Medicine![]() |
Development of the Embryo |
The development of a new human being starts with the fertilization of an ovum (egg cell) by a spermatozoon (sperm cell), resulting in the first cell of the new individual. The nuclei of the egg cell and the sperm cell contain, respectively, the maternal and paternal genes, which carry the primary instructions that guide the formation and functioning of a living being. During fertilization, these two nuclei merge, combining the maternal and paternal instructions into a unique set of genes: the genome of a new individual. The cell and all its descendants will read that genome such as a recipe; the instructions written in each gene will be carried out to generate the new living being with all its specific characteristics (Figure 1.1a).
From then on, following the instructions in its genes, the first cell divides into two, those two in four, the four into eight, and so on, until reaching the trillions of cells that make up an adult person. At each cell division, the genome formed at fertilization is copied whole and passed on to each one of the daughter cells. In this way, with the exception of end-stage egg (ovum) and sperm cells, each one of our trillions of nucleated cells contains a complete copy of our genome.
By the fourth day of development, the human embryo is an amorphous conglomerate of 16 to 30 identical cells and is not yet implanted in the uterus (Figure 1.1a). By the fifth day, the embryo contains approximately 100 cells and is called the blastocyst. At this stage of development, some cells have now developed different characteristics, and divide basically into two groups: those that will form tissues outside the embryo, such as the placenta, and those that will form the embryo itself, which is referred to as the bud. In one or two days, the blastocyst expands and implants itself in the uterus, starting a complex process of cell divisions. From that moment progressively the cells in the embryo begin to take on specific forms and functions, in a process called differentiation (Figure 1.1b).
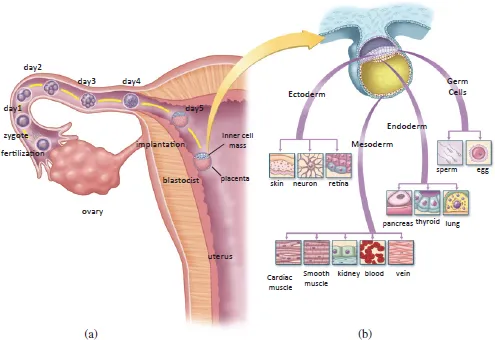
Fig. 1.1. Development of the human embryo. (a) With fertilization, the first cell (zygote) of a new individual is formed and begins to divide into identical cells. By the time it reaches the blastocyst stage, the embryo comprises two distinct cell populations: one that will give rise to the placenta and the other, the cells of the inner cell mass, that will give rise to the tissues of the new adult. (b) When the embryo cells implant in the uterus, they continue multiplying, and separate into four groups: ectoderm, mesoderm, endoderm and germ cells.
Differentiation means becoming different. Cell differentiation is the process by which otherwise identical cells become different from one another in structure and function. Remember that these few cells will give rise to all the structures in the new-born baby, from neurons and muscles through to skin, bone, liver and blood. If this process of specialization does not take place in a highly organized manner, it will not result in a normal functioning human being.
The first stage of differentiation divides the embryo into three large groups of cells called endoderm, ectoderm and mesoderm (Figure 1.1b), and a much smaller group comprising the germ cells. As the embryo develops, so the cells in each of these groups multiply and become progressively more differentiated and specialized into various tissues and organs.
The cells of the endoderm will produce the whole digestive system, liver, pancreas, kidneys and lungs. The cells of the ectoderm give rise to the skin and the nervous system, including the spinal cord and brain. Meanwhile, the cells of the mesoderm will form the connective tissues, cartilage, bones, muscles and the cardiovascular system, including the heart and blood cells. The germ cells, in the meanwhile, give rise to the gonads, either ovaries or testicles.
Initially identical cells are going to multiply and differentiate in an extremely organized fashion to produce a human being, made up of trillions of cells having more than 200 different cell and tissue types. But how do those initially identical cells acquire the specific characteristics of differentiated cells?
This process is governed by our genome, the instructions in the nucleus of each one of our cells. Thus, as the embryo develops, in some way that we do not yet fully understand, each cell begins to activate specific sets of genes, specific instructions in the genome: some will trigger particular genes to become blood cells; others, the genes of skin cells; some will turn on neuron genes, and so on (Figure 1.2). But how do the cells of the embryo know which genes to activate?
This is one of Biology’s great mysteries and, to me, one of the most fascinating. Scientists have studied it by resorting to simpler organisms, such as Drosophila melanogaster (the fruit fly) or even the millimeter-long worm Caenorhabditis elegans (C. elegans), which contains exactly 1,031 cells in the adult male. Because that worm is so simple — and is more transparent — it is easier to study its development than in higher organisms, and determine how it develops from the first cell to the adult individual.
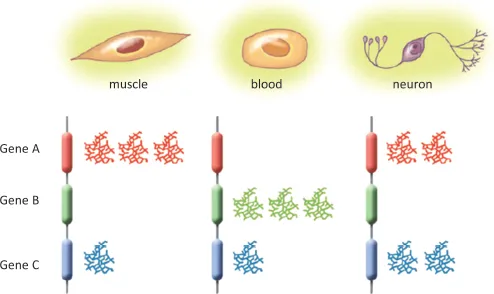
Fig. 1.2. Identity of each cell type. Nearly all the cells in your body have a nucleus containing a complete set of all your genes, a complete genome. In each type of cell, however, only one subset of those genes is activated, giving the specific characteristics of each cell type.
[Watch a video of developing C. elegans at http://www.ib.usp.br/lance.usp/booksc/video1].
But what does the development of C. elegans or Drosophila have to do with human development?
Both started with fertilization, and continued with multiplication of initially identical cells, which at some point differentiated into specialized cells. It is as if, before trying to understand how to build a 100-floor building, the budding engineer starts by studying how to build a bungalow. The same basic laws of Physics that apply there will apply also to building the skyscraper — but it is simpler to learn them with a bungalow and then go on to more complex constructions.
In the same way, basic developmental mechanisms identified in C. elegans or in Drosophila are reproduced, although in more complex form, in the development of mammals such as mice and humans. This explains how knowledge obtained in these simple experimental animal models help us to understand the development of the human embryo.
To summarize, humans are made up of trillions of cells, each one usually containing a complete copy of our genome. Each type of cell will have activated a specific set of genes, thus becoming a specialized or differentiated cell in both form and function.
![]() |
A Brief Introduction to Growing Cells Outside the Body, or Cell Culture |
With the exception of erythrocytes (red blood cells), each cell normally contains a complete genome in its nucleus. But are they able to live and multiply outside our body? Yes, but only if we provide them with the proper growth conditions, that is, nutrients, temperature and atmosphere that simulate the same environment surrounding them as inside the human body. Scientists had been researching how to do this since the 19th century, but it was not until the early 20th century that ‘cell culture’ techniques emerged. By this process it is possible to induce cells to grow outside the organism, in culture vessels in the laboratory.
Cell cultures can be set up from small fragments or aliquots of skin, blood, liver, muscle and other tissues. The fragments are placed in flasks with a liquid containing essential nutrients for growth, the “culture medium.” These flasks are then kept at a temperature of 37°C in cell incubators containing a mixture of gases (importantly CO2 and O2). In this way, the cells from tissue fragments start to multiply, grow out from the initial fragments, and spread through the flask (Figure 2.1).
In the body, blood cells live in suspension and that is also how they are grown in cell culture in flasks. Cells of solid tissues, such as skin, muscle and liver adhere to the culture flask as they grow. Each cell type requires a specific culture medium, with varying levels of sugars, proteins and other nutrients and growth factors. In fact, one of the great challenges of cell culture is how to develop a culture medium suited to growing the desired types of cells.
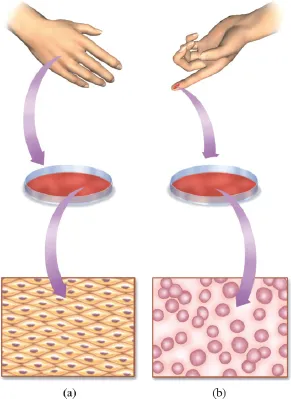
Fig. 2.1. Culturing cells in the laboratory. Human cells can be multiplied in culture flasks and observed under a microscope: (a) skin cells; (b) blood cells.
[Watch a video of cells growing from a skin biopsy specimen at http://www.ib.usp.br/lance.usp/livroct/video2].
The ability to cultivate cells in the laboratory, or in vitro, has led to great advances in basic research and medicine. Cell cultures have been used to multiply viruses to produce vaccines, against poliomyelitis, for instance. From such cultured cells, it was possible to produce important proteins, such as insulin for treatment of diabetes or antibodies to fight specific types of cancer.
In principle this means that, if cells can be multiplied in culture, the problem of demand for tissues for transplants is resolved: all you have to do is take a little piece of the desired tissue and cultivate the cells to produce enough for a transplant. Right? Well, in theory, yes; but in practice, most types of cells, such as skin cells for instance, only multiply in culture for a limited number of generations and then stop growing. The same happens with all the other tissues that have been cultivated — cells that are already differentiated and specialized multiply up to a maximum of ~60 times, then stop dividing. Worse still, some cultures that do not stop growing have generally lost the ability to control their multiplication which, in an organism, often gives rise to tumor.
Is the limitation on cultured cell proliferation a technical problem (of discovering the right conditions for cell culture) or is it a problem of biology (that normal body cells actually cannot multiply forever)? Maybe both, but we do know that the great majority of cells in the body have limited ability to multiply. However, there is a special group of non-cancer cells that can multiply almost indefinitely both in vivo and in vitro and are responsible for the normal maintenance of all organs and tissues in the human body: these are known as stem cells.
![]() | Regeneration and Stem Cells |
All through life, the body is continually replacing cells of various tissues and organs. Take blood for example: its tissues are produced continually, to the point where we can donate blood regularly — we produce some 100 billion new blood cells every day! Al...
Table of contents
- Cover
- Halftitle
- Title
- Copyright
- Contents
- Introduction — In Search of Eternal Life
- Acknowledgments
- Chapter 1 Development of the Embryo
- Chapter 2 A Brief Introduction to Growing Cells Outside the Body, or Cell Culture
- Chapter 3 Regeneration and Stem Cells
- Chapter 4 Adult Stem Cells
- Chapter 5 Embryonic Stem Cells
- Chapter 6 Cell Therapy — Promise or Reality?
- Chapter 7 Outlook
- Final Note
- References
- Index