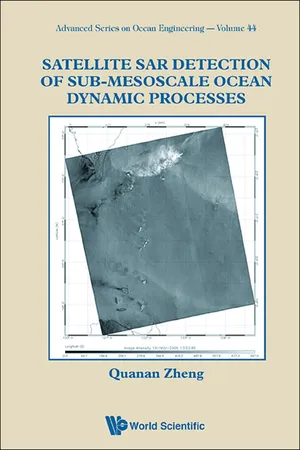
- 348 pages
- English
- ePUB (mobile friendly)
- Available on iOS & Android
Satellite SAR Detection of Sub-Mesoscale Ocean Dynamic Processes
About This Book
-->
Synthetic-aperture radar (SAR) as a form of radar to create images of objects, uses the motion of the radar antenna over a targeted region to provide finer spatial resolution than is possible with conventional beam-scanning radars by mounting the antenna on a moving platform such as an aircraft or spacecraft. As antenna aperture (the "size" of the antenna) is defined by the distance the SAR device travels over a target in the time taken for the radar pulses to return to the antenna, the larger the aperture is, the higher the image resolution, therefore, this enables SAR to create high resolution images with comparatively small physical antennas.
This special book aims to provide the updated theories and methods for the use of synthetic aperture radar (SAR) onboard satellites to detect ocean processes, i.e., SAR ocean remote sensing. It is a hi-tech application field having been developed since late 1970s and become a powerful tool for obtaining dynamic signatures from the remote and broad ocean.
--> Contents:
- Principles of Radar Imaging of Ocean Processes
- Sub-Mesoscale Ocean Vortex Train
- Spirals on the Sea
- Ocean Near-Inertial Oscillations
- Estuary Outflow Jet
- Ocean Internal Waves: I. Dynamics and Parameter Extraction
- Ocean Internal Waves: II. Statistical Analysis
- Ocean Bottom Topography: I. Longitudinal Flow Passing Over Parallel Topographic Corrugations
- Ocean Bottom Topography: II. Traverse Flow Passing Over Periodical Topography
- Ocean Bottom Topography: III. Stratified Flow Passing Over Isolated Topography
- Marine Atmospheric Boundary Layer Processes
--> -->
Readership: Graduate, academics and professionals in the field of satellite oceanography, ocean engineering, geophysics, electrical and electronic engineering, and earth science.
-->Keywords:Satellite SAR;Sub-Mesoscale Ocean Processes;Ocean Internal Waves;Ocean Bottom Topography;Air-Sea Boundary ProcessesReview: Key Features:
- Differing from general ocean remote sensing books, this book focuses on one subject only, i.e., SAR detection of the ocean. Thus it is more specified and more concentrated. The fundamental material for the book is the research results obtained by the author during the last two decades and also includes the contributions from other investigators
Frequently asked questions
Information
Chapter 1
Principles of Radar Imaging of Ocean Processes
1.1.Radar Principles
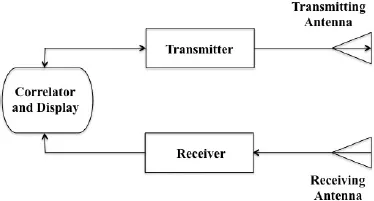


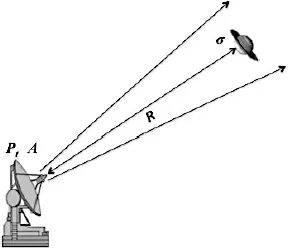




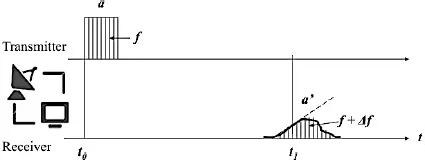
Table of contents
- Cover Page
- Title
- Copyright
- Contents
- Preface
- List of Tables
- List of Figures
- Chapter 1. Principles of Radar Imaging of Ocean Processes
- Chapter 2. Sub-Mesoscale Ocean Vortex Train
- Chapter 3. Spirals on the Sea
- Chapter 4. Ocean Near-Inertial Oscillations
- Chapter 5. Estuary Outflow Jet
- Chapter 6. Ocean Internal Waves: I. Dynamics and Parameter Extraction
- Chapter 7. Ocean Internal Waves: II. Statistical Analysis
- Chapter 8. Ocean Bottom Topography: I. Longitudinal Flow Passing Over Parallel Topographic Corrugations
- Chapter 9. Ocean Bottom Topography: II. Traverse Flow Passing Over Periodical Topography
- Chapter 10. Ocean Bottom Topography: III. Stratified Flow Passing Over Isolated Topography
- Chapter 11. Marine Atmospheric Boundary Layer Processes
- Bibliography
- Index