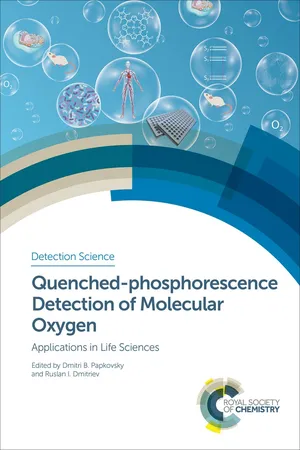
Quenched-phosphorescence Detection of Molecular Oxygen
Applications in Life Sciences
- 368 pages
- English
- ePUB (mobile friendly)
- Available on iOS & Android
Quenched-phosphorescence Detection of Molecular Oxygen
Applications in Life Sciences
About This Book
Significant progress has been made in recent years in quenched-phosphorescence oxygen sensing, particularly in the materials and applications of this detection technologythat are open to commercialization, like uses in brain imaging and food packaging. Prompted by this, the editors have delivered a dedicated bookthat brings together these developments, provides a comprehensive overview of the different detection methodologies, and representative examples and applications.
This book is intended to attract new researchers from various disciplines such as chemistry, physics, biology and medicine, stimulate further progress in the field and assist in developing new applications. Providing a concise summary at the cutting edge, this practical guide for current experts and new potential users will increase awareness of this versatile sensing technology.
Frequently asked questions
Information
*E-mail: [email protected]
1.1 Introduction
1.2 Mechanism of Oxygen Quenching
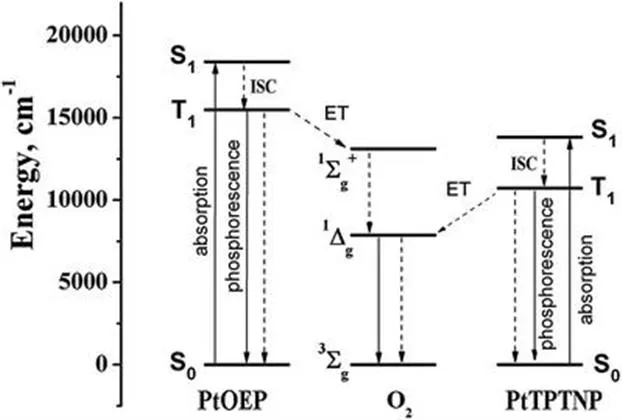

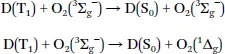
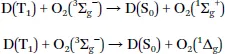


1.3 Requirements for Phosphorescent Indicators
- (i) Spectral properties (absorption and emission maxima). In contrast to fluorescent dyes, phosphorescent indicators possess large Stokes shifts, which simplifies signal separation and reduces interferences caused by scattering and autofluorescence. Nevertheless, indicators excitable and emitting at longer wavelength are preferable for the same reasons. In photosynthetic systems, however, such excitation can result in much higher levels of autofluorescence and dyes with other spectral properties can be a better choice. Clearly, autofluorescence can be completely eliminated in the time domain measurement, but it does interfere with the measurement in the frequency domain unless multi-frequency measurement is performed. Compatibility of the indicator with the light sources, photodetectors and other optical components should also be considered. Whereas a wide range of light sources is available for the whole spectral range, the detectors are mainly limited to avalanche photodiodes, CCD-arrays and photomultipliers. Although the sensitivity of PMTs is generally very high, it deteriorates fast in the NIR part of the spectrum. In the case of fiber-optic sensors, the quartz glass fibers are compatible with all oxygen indicators. In contrast, much cheaper plastic fibers show strong absorption in the NIR part of the spectrum, which limits the practically useful length to 1ā2 meters.
- (ii) Brightness of luminescence. Brightness can be defined as the product of molar absorption coefficient ε and luminescence quantum yield Ļ. Clearly, in case of phosphorescent indicators high efficiency of inter-system crossing (ISC, S1 ā T1 transition, Figure 1.1) is one of the prerequisites for bright phosphorescence. Bright indicators allow for thinner sensing layers and therefore for faster response times. In the case of very bright but less photostable indicators the oper...
Table of contents
- Cover
- Title
- Preface
- Contents
- Chapter 1 Fundamentals of Quenched Phosphorescence O2 Sensing and Rational Design of Sensor Materials
- Chapter 2 New Polymer-based Sensor Materials and Fabrication Technologies for Large-scale Applications
- Chapter 3 Evolution of Cell-penetrating Phosphorescent O2 Probes
- Chapter 4 Hydrophilic Ir(iii) Complexes for In vitro and In vivo Oxygen Imaging
- Chapter 5 Protection of Triplet Excited State Materials from Oxygen Quenching and Photooxidation in Optical Sensing Applications
- Chapter 6 Progress in Phosphorescence Lifetime Measurement Instrumentation for Oxygen Sensing
- Chapter 7 Optical O2 Sensing in Aquatic Systems and Organisms
- Chapter 8 Monitoring of Extracellular and Intracellular O2 on a Time-resolved Fluorescence Plate Reader
- Chapter 9 Monitoring Parameters of Oxygen Transport to Cells in the Microcirculation
- Chapter 10 Photoacoustic Imaging of Oxygen
- Chapter 11 Imaging of Tissue Oxygen Ex vivo
- Chapter 12 Tracking of Hypoxia and Cancer Metastasis with Iridium(iii)-based O2 Probes
- Chapter 13 Probing Tissue Oxygenation by Delayed Fluorescence of Protoporphyrin IX
- Chapter 14 Microfluidic Systems and Optical Oxygen Sensors: A Perfect Match for Advancing Bioprocessing and Microbiology
- Chapter 15 pO2 Measurements in Biological Tissues by Luminescence Lifetime Spectroscopy: Strategies to Exploit or Minimize Phototoxic Effects in Tumors
- Chapter 16 In vivo Brain Functional Imaging Using Oxygenation-related Optical Signal
- Chapter 17 Applications of Phosphorescent O2 Sensors in Food and Beverage Packaging Systems
- Subject Index