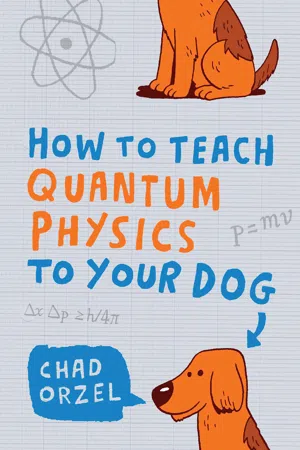
This is a test
- 304 pages
- English
- ePUB (mobile friendly)
- Available on iOS & Android
eBook - ePub
How to Teach Quantum Physics to Your Dog
Book details
Book preview
Table of contents
Citations
About This Book
The international bestseller from the author of Breakfast with Einstein Emmy is no ordinary dog. When adopted from the shelter by physics professor Chad Orzel, she becomes immediately fascinated by his work. Could she use quantum tunnelling to get through the neighbour's fence? How about diffracting round a tree to chase squirrels? Or using virtual particles to catch bunnies made of cheese?Taking Emmy's anarchic behaviour as a starting point, Orzel explains the key theories of quantum physics. From quarks and gluons to Heisenberg's uncertainty principle, this is a uniquely entertaining way to unlock the secrets of the universe.
Frequently asked questions
At the moment all of our mobile-responsive ePub books are available to download via the app. Most of our PDFs are also available to download and we're working on making the final remaining ones downloadable now. Learn more here.
Both plans give you full access to the library and all of Perlegoâs features. The only differences are the price and subscription period: With the annual plan youâll save around 30% compared to 12 months on the monthly plan.
We are an online textbook subscription service, where you can get access to an entire online library for less than the price of a single book per month. With over 1 million books across 1000+ topics, weâve got you covered! Learn more here.
Look out for the read-aloud symbol on your next book to see if you can listen to it. The read-aloud tool reads text aloud for you, highlighting the text as it is being read. You can pause it, speed it up and slow it down. Learn more here.
Yes, you can access How to Teach Quantum Physics to Your Dog by Chad Orzel in PDF and/or ePUB format, as well as other popular books in Physical Sciences & Quantum Theory. We have over one million books available in our catalogue for you to explore.
Information
Topic
Physical SciencesSubtopic
Quantum TheoryCHAPTER 1
Which Way? Both Ways:
Particle-Wave Duality
Particle-Wave Duality
Weâre out for a walk, when the dog spots a squirrel up ahead and takes off in pursuit. The squirrel flees into a garden and dodges around a small ornamental maple tree. Emmy doesnât alter her course in the slightest, and just before she slams into the tree, I pull her up short.
âWhatâd you do that for?â she asks, indignantly.
âWhat do you mean? You were about to run into a tree, and I stopped you.â
âNo I wasnât.â She looks off after the squirrel, now safely up a bigger tree on the other side of the garden. âBecause of quantum.â
We start walking again. âOkay, youâre going to have to explain that,â I say.
âWell, I have this plan,â she says. âYou know how when I chase the bunnies in the back garden, when I run to the right of the pond, they go left, and get away?â
âYes.â
âAnd when I run to the left of the pond, they go right, and get away?â
âYes.â
âWell, Iâve thought of a new way to run, so they canât escape.â
âWhat, through the middle of the pond?â Itâs only about eight inches deep and a couple of feet across.
âNo, silly. Iâm going to go both ways. Iâll trap the bunnies between me.â
âRight ⊠Thatâs an ⊠interesting theory.â
âItâs not a theory, itâs quantum physics. Material particles have wave nature and can diffract around objects. If you send a beam of electrons at a barrier, theyâll go around it to the left and to the right, at the same time.â Sheâs really getting into this, and she doesnât even notice the cat sunning itself in the garden across the street. âSo, Iâll just make use of my wave nature, and go around both sides of the pond.â
âAnd where does running headfirst into a tree come in?â
âOh, well.â She looks a little sheepish. âI thought I would try it out on something smaller first. I got a good running start, and I was just about to go around when you stopped me.â
âAh. Like I said, an interesting theory. It wonât work, you know.â
âYouâre not going to try to claim I donât have wave nature, are you? Because I do. Itâs in your physics books.â
âNo, no, youâve got wave nature, all right. Youâve also got Buddha natureââ
âIâm an enlightened dog!â
ââwhich will do you about as much good. You see, a tree is big, and your wavelength is small. At walking speed, a twenty-kilogram dog like you has a wavelength of about 10-35 meters. You need your wavelength to be comparable to the size of the treeâmaybe ten centimetersâin order to diffract around it, and youâre thirty-four orders of magnitude off.â
âIâll just change my wavelength by changing my momentum. I can run very fast.â
âNice try, but the wavelength gets shorter as you go faster. To get your wavelength up to the millimeter or so youâd need to diffract around a tree, youâd have to be moving at 10-30 meters per second, and thatâs impossibly slow. It would take a billion years to cross the nucleus of an atom at that speed, which is far too slow to catch a bunny.â
âSo, youâre saying I need a new plan?â
âYou need a new plan.â
Her tail droops, and we walk in silence for a few seconds. âRight,â she says, âcan you help me with my new plan?â
âI can try.â
âHow do I use my Buddha nature to go around both sides of the pond at the same time?â
I really canât think of anything to say to that, but a flash of gray fur saves me. âLook! A squirrel!â I say.
And weâre off in pursuit.
Quantum physics has many strange and fascinating aspects, but the discovery that launched the theory was particle-wave duality, or the fact that both light and matter have particle-like and wavelike properties at the same time. A beam of light, which is generally thought of as a wave, turns out to behave like a stream of particles in some experiments. At the same time, a beam of electrons, which is generally thought of as a stream of particles, turns out to behave like a wave in some experiments. Particle and wave properties seem to be contradictory, and yet everything in the universe somehow manages to be both a particle and a wave.
The discovery in the early 1900s that light behaves like a particle is the launching point for all of quantum mechanics. In this chapter, weâll describe the history of how physicists discovered this strange duality. In order to appreciate just what a strange development this is, though, we need to talk about the particles and waves that we see in everyday life.
PARTICLES AND WAVES AROUND YOU: CLASSICAL PHYSICS
Everybody is familiar with the behavior of material particles. Pretty much all the objects you see around youâbones, balls, squeaky toysâbehave like particles in the classical sense, with their motion determined by classical physics. They have different shapes, but you can predict their essential motion by imagining each as a small, featureless ball with some massâa particleâand applying Newtonâs laws of motion.* A tennis ball and a long bone tumbling end over end look very different in flight, but if theyâre thrown in the same direction with the same speed, theyâll land in the same place, and you can predict that place using classical physics.
A particle-like object has a definite position (you know right where it is), a definite velocity (you know how fast itâs moving, and in what direction), and a definite mass (you know how big it is). You can multiply the mass and velocity together, to find the momentum. A great big Labrador retriever has more momentum than a little French poodle when theyâre both moving at the same speed, and a fast-moving border collie has more momentum than a waddling basset hound of the same mass. Momentum determines what will happen when two particles collide. When a moving object hits a stationary one, the moving object will slow down, losing momentum, while the stationary object will speed up, gaining momentum.
The other notable feature of particles is something that seems almost too obvious to mention: particles can be counted. When you have some collection of objects, you can look at them and determine exactly how many of them you haveâone bone, two squeaky toys, three squirrels under a tree in the back garden.
Waves, on the other hand, are slipperier. A wave is a moving disturbance in something, like the patterns of crests and troughs formed by water splashing in a garden pond. Waves are spread out over some region of space by their nature, forming a pattern that changes and moves over time. No physical objects move anywhereâthe water stays in the pondâbut the pattern of the disturbance changes, and we see that as the motion of a wave.
If you want to understand a wave, there are two ways of looking at it that provide useful information. One is to imagine taking a snapshot of the whole wave, and looking at the pattern of the disturbance in space. For a single simple wave, you see a pattern of regular peaks and valleys, like this:
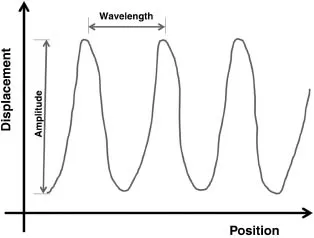
As you move along the pattern, you see the medium moving up and down by an amount called the âamplitudeâ of the wave. If you measure the distance between two neighboring crests of the wave (or two troughs), youâve measured the âwavelength,â which is one of the numbers used to describe a wave.
The other thing you can do is to look at one little piece of the wave pattern, and watch it for a long timeâimagine watching a duck bobbing up and down on a lake, say. If you watch carefully, youâll see that the disturbance gets bigger and smaller in a very regular wayâsometimes the duck is higher up, sometimes lower downâand makes a pattern in time very much like the pattern in space. You can measure how often the wave repeats itself in a given amount of timeâhow many times the duck reaches its maximum height in a minute, sayâand that gives you the âfrequencyâ of the wave, which is another critical number used to describe the wave. Wavelength and frequency are related to each otherâlonger wavelengths mean lower frequency, and vice versa.
You can already see how waves are different from particles: they donât have a position. The wavelength and the frequency describe the pattern as a whole, but thereâs no single place you can point to and identify as the position of the wave. The wave itself is a disturbance spread over space, and not a physical thing with a definite position and velocity. You can assign a velocity to the wave pattern, by looking at how long it takes one crest of the wave to move from one position to another, but again, this is a property of the pattern as a whole.
You also canât count waves the way you can count particlesâyou can say how many crests and troughs there are in one particular area, but those are all part of a single wave pattern. Waves are continuous where particles are discreteâyou can say that you have one, two, or three particles, but you either have waves, or you donât. Individual waves may have larger or smaller amplitudes, but they donât come in chunks like particles do. Waves donât even add together in the same way that particles doâsometimes, when you put two waves together, you end up with a bigger wave, and sometimes you end up with no wave at all.
Imagine that you have two different sources of waves in the same areaâtwo rocks thrown into still water at the same time, for example. What you get when you add the two waves together depends on how they line up. If you add the two waves together such that the crests of one wave fall on top of the crests of the other, and the troughs of one wave fall in the troughs of the other (such waves are called âin phaseâ), youâll get a larger wave than either of the two you started with. On the other hand, if you add two waves together such that the crests of one wave fall in the troughs of the other and vice versa (âout of phaseâ), the two will cancel out, and youâll end up with no wave at all.
This phenomenon is called interference, and itâs perhaps the most dramatic difference between waves and particles.
âI donât know ⊠thatâs pretty weird. Do you have any other examples of interference? Something more ⊠doggy?â
âNo, I really donât. Thatâs the pointâwaves are dramatically different than particles. Nothing that dogs deal with on a regular basis is all that wavelike.â
âHow about, âInterference is like when you put a squirrel in the garden, and then you put a dog in the garden, and a minute later, thereâs no more squirrel in the garden.â â
âThatâs not interference, thatâs prey pursuit. Interference is more like putting a squirrel in the garden, then putting a second squirrel in the garden one second later, and finding that you have no squirrels at all. But if you wait two seconds before putting in the second squirrel, you find four squirrels.â
âOkay, thatâs just weird.â
âThatâs my point.â
âOh. Well, good job, then. Anyway, why are we talking about this?â
âWell, you need to know a few things about waves in order to understand quantum physics.â
âYes, but this just sounds like maths. I donât like maths. When are we going to talk about physics?â
âWe are talking about physics. The whole point of physics is to use maths to describe the universe.â
âI donât want to describe the universe, I want to catch squirrels.â
âWell, if you know how to describe the universe with maths, that can help you catch squirrels. If you have a mathematical model of where the squirrels are now, and you know the rules governing squirrel behavior, you can use your model to predict where theyâll be later. And if you can predict where theyâll be later âŠâ
âI can catch squirrels!â
âExactly.â
âAll right, maths is okay. I still donât see what this wave stuff is for, though.â
âWe need it to explain the properties of light and sound waves, which is the next bit.â
WAVES IN EVERYDAY LIFE: LIGHT AND SOUND
We deal with two kinds of waves in everyday life: light and sound. Though these are both examples of wave phenomena, they appear to behave very differently. The reasons for those differences will help shed some light (pardon the pun) on why it is that we donât see dogs passing around both sides of a tree at the same time.
Sound waves are pressure waves in the air. When a dog barks, she forces air out through her mouth and sets up a vibration that travels through the air in all directions. When it reaches another dog, that sound wave causes vibrations in the second dogâs eardrums, which are turned into signals in the brain that are processed as sound, causing the second dog to bark, producing more waves, until nearby humans get annoyed.
Light is a different kind of wave, an oscillating electric and magnetic field that travels through spaceâeven the emptiness of outer space, which is why we can see distant stars and galaxies. When light waves strike the back of your eye, they get turned into signals in the brain that are processed to form an image of the world around you.
The most striking difference between light and sound in everyday life has to do with what happens when they encounter an obstacle. Light waves travel only in straight lines, while sound waves seem to bend around obstacles. This is why a dog in the dining room can hear a potato chip hitting the kitchen floor, even though she canât see it.
The apparent bending of sound waves around corners is an example of diffraction, which is a characteristic behavior of waves encountering an obstacle. When a wave reaches a barrier with an opening in it, like th...
Table of contents
- Cover
- Title Page
- Dedication
- Contents
- Introduction
- Chapter 1: Which Way? Both Ways: Particle-Wave Duality
- Chapter 2: Whereâs My Bone? The Heisenberg Uncertainty Principle
- Chapter 3: Schrödingerâs Dog: The Copenhagen Interpretation
- Chapter 4: Many Worlds, Many Treats: The Many-Worlds Interpretation
- Chapter 5: Are We There Yet? The Quantum Zeno Effect
- Chapter 6: No Digging Required: Quantum Tunneling
- Chapter 7: Spooky Barking at a Distance: Quantum Entanglement
- Chapter 8: Beam Me a Bunny: Quantum Teleportation
- Chapter 9: Bunnies Made of Cheese: Virtual Particles and Quantum Electrodynamics
- Chapter 10: Beware of Evil Squirrels: Misuses of Quantum Physics
- Acknowledgments
- Further Reading
- Glossary of Important Terms
- Index
- Copyright