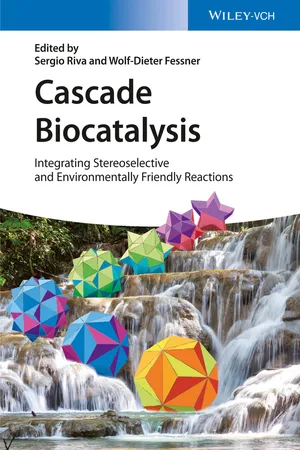
Cascade Biocatalysis
Integrating Stereoselective and Environmentally Friendly Reactions
- English
- ePUB (mobile friendly)
- Available on iOS & Android
Cascade Biocatalysis
Integrating Stereoselective and Environmentally Friendly Reactions
About This Book
This ready reference presents environmentally friendly and stereoselective methods of modern biocatalysis. The experienced and renowned team of editors have gathered top international authors for this book. They cover such emerging topics as chemoenzymatic methods and multistep enzymatic reactions, while showing how these novel methods and concepts can be used for practical applications. Multidisciplinary topics, including directed evolution, dynamic kinetic resolution, and continuous-flow methodology are also discussed. From the contents:
* Directed Evolution of Ligninolytic Oxidoreductases: from Functional Expression to Stabilization and Beyond
* New Trends in the In Situ Enzymatic Recycling of NAD(P)(H) Cofactors
* Monooxygenase-Catalyzed Redox Cascade Biotransformations
* Biocatalytic Redox Cascades Involving w-Transaminases
* Multi-Enzyme Systems and Cascade Reactions Involving Cytochrome P450 Monooxygenases
* Chemo-Enzymatic Cascade Reactions for the Synthesis of Glycoconjugates
* Synergies of Chemistry and Biochemistry for the Production of Beta-Amino Acids
* Racemizable Acyl Donors for Enzymatic Dynamic Kinetic Resolution
* Stereoselective Hydrolase-Catalyzed Processes in Continuous-Flow Mode
* Perspectives on Multienzyme Process Technology
* Nitrile Converting Enzymes Involved in Natural and Synthetic Cascade Reactions
* Mining Genomes for Nitrilases
* Key-Study on the Kinetic Aspects of the In-Situ NHase/AMase Cascade System of M. imperiale Resting Cells for Nitrile Bioconversion
* Enzymatic Stereoselective Synthesis of Beta-Amino Acids
* New Applications of Transketolase: Cascade Reactions for Assay Development
* Aldolases as Catalyst for the Synthesis of Carbohydrates and Analogs
* Enzymatic Generation of Sialoconjugate Diversity
* Methyltransferases in Biocatalysis
* Chemoenzymatic Multistep One-Pot Processes
Frequently asked questions
Information
Chapter 1
Directed Evolution of Ligninolytic Oxidoreductases: from Functional Expression to Stabilization and Beyond
1.1 Introduction
1.2 Directed Molecular Evolution
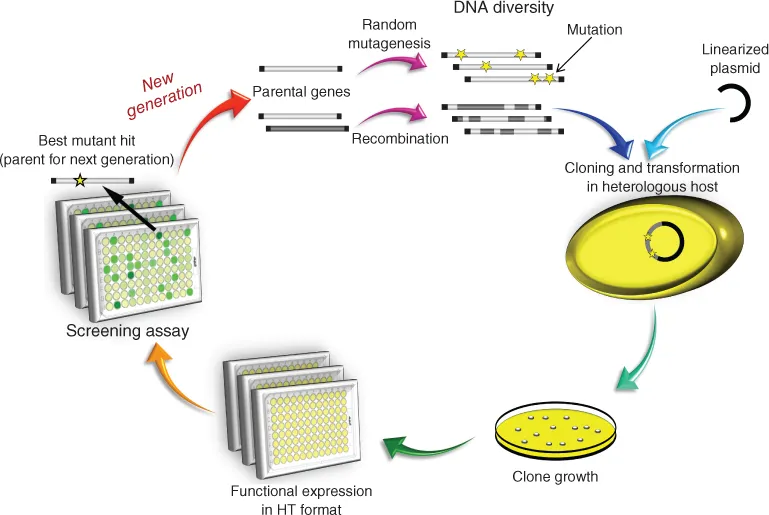
1.3 The Ligninolytic Enzymatic Consortium
Table of contents
- Cover
- Related Titles
- Title Page
- Copyright
- List of Contributors
- Preface
- Chapter 1: Directed Evolution of Ligninolytic Oxidoreductases: from Functional Expression to Stabilization and Beyond
- Chapter 2: New Trends in the In Situ Enzymatic Recycling of NAD(P)(H) Cofactors
- Chapter 3: Monooxygenase-Catalyzed Redox Cascade Biotransformations
- Chapter 4: Biocatalytic Redox Cascades Involving Ď-Transaminases
- Chapter 5: Multi-Enzyme Systems and Cascade Reactions Involving Cytochrome P450 Monooxygenases
- Chapter 6: Chemo-Enzymatic Cascade Reactions for the Synthesis of Glycoconjugates
- Chapter 7: Synergies of Chemistry and Biochemistry for the Production of β-Amino Acids
- Chapter 8: Racemizable Acyl Donors for Enzymatic Dynamic Kinetic Resolution
- Chapter 9: Stereoselective Hydrolase-Catalyzed Processes in Continuous-Flow Mode
- Chapter 10: Perspectives on Multienzyme Process Technology
- Chapter 11: Nitrile Converting Enzymes Involved in Natural and Synthetic Cascade Reactions
- Chapter 12: Mining Genomes for Nitrilases
- Chapter 13: Key-Study on the Kinetic Aspects of the In Situ NHase/AMase Cascade System of M. imperiale Resting Cells for Nitrile Bioconversion
- Chapter 14: Enzymatic Stereoselective Synthesis of β-Amino Acids
- Chapter 15: New Applications of Transketolase: Cascade Reactions for Assay Development
- Chapter 16: Aldolases as Catalyst for the Synthesis of Carbohydrates and Analogs
- Chapter 17: Enzymatic Generation of Sialoconjugate Diversity
- Chapter 18: Methyltransferases in Biocatalysis
- Chapter 19: Chemoenzymatic Multistep One-Pot Processes
- Index
- End User License Agreement