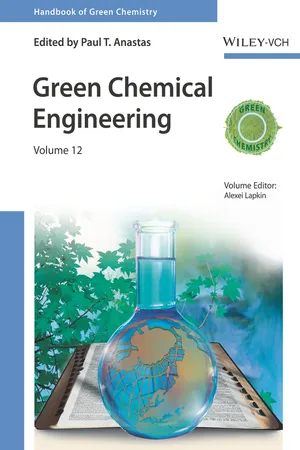
This is a test
- English
- ePUB (mobile friendly)
- Available on iOS & Android
eBook - ePub
Green Chemical Engineering, Volume 12
Book details
Book preview
Table of contents
Citations
About This Book
Green chemistry and chemical engineering belong together and this twelth volume in the successful Handbook of Green Chemistry series represents the perfect one-stop reference on the topic.
Written by an international team of specialists with each section edited by international leading experts, this book provides first-hand insights into the field, covering chemical engineering process design, innovations in unit operations and manufacturing, biorefining and much more besides.
An indispensable source for every chemical engineer in industry and academia.
Frequently asked questions
At the moment all of our mobile-responsive ePub books are available to download via the app. Most of our PDFs are also available to download and we're working on making the final remaining ones downloadable now. Learn more here.
Both plans give you full access to the library and all of Perlego’s features. The only differences are the price and subscription period: With the annual plan you’ll save around 30% compared to 12 months on the monthly plan.
We are an online textbook subscription service, where you can get access to an entire online library for less than the price of a single book per month. With over 1 million books across 1000+ topics, we’ve got you covered! Learn more here.
Look out for the read-aloud symbol on your next book to see if you can listen to it. The read-aloud tool reads text aloud for you, highlighting the text as it is being read. You can pause it, speed it up and slow it down. Learn more here.
Yes, you can access Green Chemical Engineering, Volume 12 by Paul T. Anastas, Paul T. Anastas in PDF and/or ePUB format, as well as other popular books in Physical Sciences & Environmental Science. We have over one million books available in our catalogue for you to explore.
Information
1
Chemical Engineering Science and Green Chemistry – The Challenge of Sustainability
Alexei A. Lapkin
1.1 Sustainability Challenge for the Chemical Industry
The challenge of sustainability is well illustrated by the global ecological footprint “wedge” (Figure 1.1). It shows that the global footprint is exceeding the ecological reserve since 1960s [1]. Footprint is only one aspect of sustainability, reflecting the use and availability of resources and damage to natural environment. The sustainability challenge could be paraphrased as achieving economic prosperity, while ensuring social equality and well-being and not destroying the environment [2]. The ecological footprint is one of the more critical aspects for today: it reflects several important relationships that must be understood in order to identify the path to feasible solutions of the sustainability challenge. The key relationship is between human aspirations and our ways of attaining them. Here, we will not go into the topic of aspirations. This is discussed in detail in sustainability literature and includes satisfaction of basic needs, aspirations of a certain standard of living, aspirations toward self-fulfillment and self-realization, which are highly context specific. What is important for us is that there appears to be a clear positive link between the standard of living and the energy intensity per capita. This ultimately stems from two factors: (i) the way we attain the increases in our standard of living and (ii) basic thermodynamics. Our standard of living today depends on access to manufactured goods used in production of building materials, clothing, food, modern education and entertainment technology, modern healthcare, and so on. In turn, manufacturing necessarily involves expenditure of energy, as we transform matter into more complex and ordered forms [3]. As population grows and a larger proportion of the population increases its standard of living, the demand for energy and resources will grow, due to the link between the standard of living and the energy required to achieve it. At present, the developed countries with a high human development index (HDI) [4] and the ecological footprint far exceeding their own resources, export their waste to, and import resources from, the countries with low HDI and low ecological footprint. This leads to inequality and exploitation, and this situation of course cannot continue indefinitely.
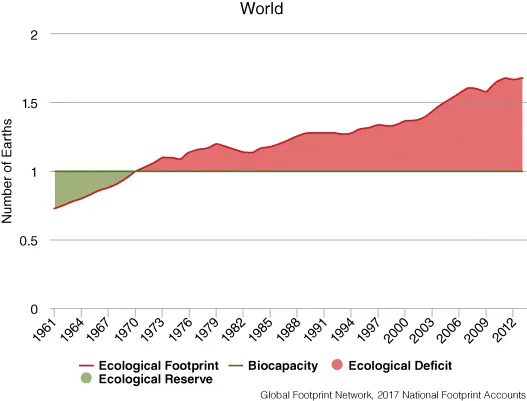
Figure 1.1 A comparison of ecological footprint, represented as a number of Earths, versus biocapacity. (Global Footprint Network [5].)
The second reason why it is critical to decouple HDI from energy demand is the apparent anthropogenic effect on climate. The vary rapid increase in global population of humans and their aspirations toward better life result in a rapidly increasing demand for energy [6–8]. At present, this results in the increase in the rate of emissions of carbon dioxide that today far exceeds the rate of biological and geological sequestration of atmospheric carbon [9], resulting in the observed climate change.
The fundamental challenge of sustainability, therefore, is to decouple the quality of life and human aspirations from energy and material intensities of achieving them.
In this respect, chemical industry will play a significant role. Chemistry is ubiquitous in everyday life and is responsible for the majority of innovations in all aspects of life, from agriculture and food to healthcare, sport, entertainment, and fashion. It is also the major source of innovation in the energy-efficient technologies. Calculation of the environmental impact of the manufacture of materials for energy generation and energy-efficient technologies, and comparison with the saved emissions by these technologies (Figure 1.2) shows the significance of chemistry to finding ways of decoupling HDI and ecological footprint. These data focus on carbon emissions, which today are regarded as the most important target as the driver of climate change [7]. Among the larger contributions to the reduction of carbon emissions by innovation in chemistry are development of insulation materials and materials for construction of wind turbines; fuel technology, including fuel additives for better combustion; shift to lower carbon fuels; novel materials for energy-efficient lighting, and detergents for low-temperature cleaning. Thus, the manufacture of novel materials is an important technological solution for reduction of carbon emissions, as it directly affects two of the major anthropogenic sources of carbon emissions, namely, transport and housing. Of course, the manufacture of materials is, basically, chemistry.
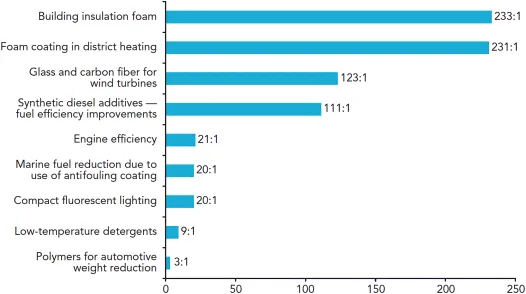
Figure 1.2 Examples of the green house gas emission savings enabled by chemistry, represented as a ratio of emission savings to emissions incurred. (Adapted from Refs [10, 11].)
These solutions are not affecting the way how energy is being produced, but are directed at efficiency of the use of energy: A reduction in the waste of energy should result in lowering the demand for energy production. The last decade saw a rapid uptake of renewable energy technologies, in particular wind and solar power. As a result, a new energy technology paradigm has emerged – chemistry as a major industrial energy storage system (Figure 1.3). This paradigm is based on the proposition that excess power will be available from renewable energy installations at low-demand off-peak times during a day. This low-cost electricity could be used to convert unreactive molecules, CO2 and H2O, into basic chemical feedstocks, such as methanol and olefins, in what has been termed “CO2 recycling technology” [12]. In a way this approach parallels the biological conversion of carbon dioxide into molecules of higher exergy [13], driven by constant supply of solar energy, but at a higher intensity (defined as production rate per unit area).
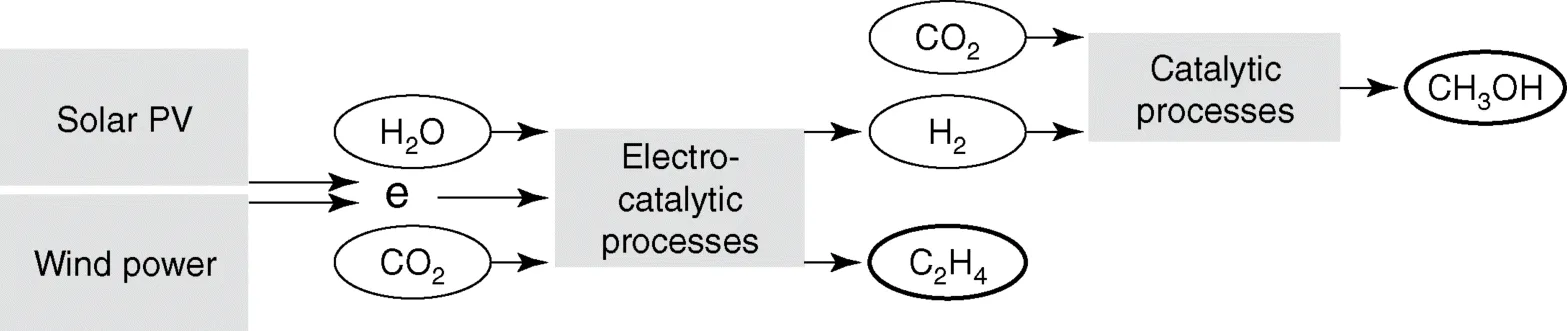
Figure 1.3 Chemical industry as an energy storage solution.
An estimate of the potential scale of the CO2 recycling technology has been given for the example of using the potentially available electricity to synthesize methanol from CO2: This technology can produce 1.2 × 109 metric tones of methanol from CO2 per year [12]. This is an order of magnitude larger amount than the global methanol production via current conventional technology. Of course, in this case the CO2-derived methanol is not a final product, but a convenient to transport source of an activated C1 group for further conversion to bulk chemical products. This technology may have a very significant impact on the overall chemical supply chain and, consequently, reduce emissions from the manufacture of chemicals.
To understand the impact of CO2 recycling technology on global emissions, it is necessary to compare life cycle impacts from conventional routes to methanol, with the proposed CO2-based technologies. The global warming potential or green house gas (GHGs) emissions, evaluated in the units of kilogram CO2 equivalents per kilogram of product, is one of the most important indicators. The total contribution of the CO2 recycling technology to GHG is comprised of three main contributions: direct use of CO2 as a feedstock, avoiding CO2 emissions from conventional routes to methanol, and CO2 emissions due to the new processes. These values would vary depending on the CO2 recycling technology used. As an example, the estimate of G...
Table of contents
- Cover
- Related Titles
- Title Page
- Copyright
- About the Editors
- List of Contributors
- Preface
- Chapter 1: Chemical Engineering Science and Green Chemistry – The Challenge of Sustainability
- Part One: Molecular Engineering of Materials, Reactions, and Processes
- Part Two: Innovations in Design, Unit Operations, and Manufacturing
- Part Three: Enabling Technologies
- Index
- End User License Agreement