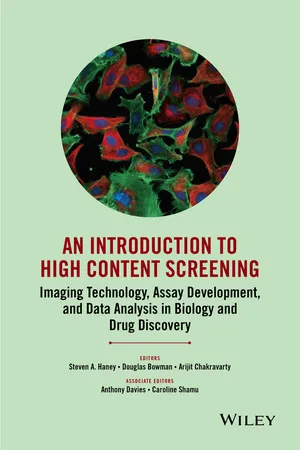
eBook - ePub
An Introduction To High Content Screening
Imaging Technology, Assay Development, and Data Analysis in Biology and Drug Discovery
This is a test
- English
- ePUB (mobile friendly)
- Available on iOS & Android
eBook - ePub
An Introduction To High Content Screening
Imaging Technology, Assay Development, and Data Analysis in Biology and Drug Discovery
Book details
Book preview
Table of contents
Citations
About This Book
Using a collaborative and interdisciplinary author base with experience in the pharmaceutical industry and academia, this book is a practical resource for high content (HC) techniques.
â˘Instructs readers on the fundamentals of high content screening (HCS) techniques
â˘Focuses on practical and widely-used techniques like image processing and multiparametric assays
â˘Breaks down HCS into individual modules for training and connects them at the end
â˘Includes a tutorial chapter that works through sample HCS assays, glossary, and detailed appendices
Frequently asked questions
At the moment all of our mobile-responsive ePub books are available to download via the app. Most of our PDFs are also available to download and we're working on making the final remaining ones downloadable now. Learn more here.
Both plans give you full access to the library and all of Perlegoâs features. The only differences are the price and subscription period: With the annual plan youâll save around 30% compared to 12 months on the monthly plan.
We are an online textbook subscription service, where you can get access to an entire online library for less than the price of a single book per month. With over 1 million books across 1000+ topics, weâve got you covered! Learn more here.
Look out for the read-aloud symbol on your next book to see if you can listen to it. The read-aloud tool reads text aloud for you, highlighting the text as it is being read. You can pause it, speed it up and slow it down. Learn more here.
Yes, you can access An Introduction To High Content Screening by Steven A. Haney, Douglas Bowman, Arijit Chakravarty, Steven A. Haney, Douglas Bowman, Arijit Chakravarty in PDF and/or ePUB format, as well as other popular books in Biological Sciences & Biochemistry. We have over one million books available in our catalogue for you to explore.
Information
1
INTRODUCTION
STEVEN A. HANEY
1.1 THE BEGINNING OF HIGH CONTENT SCREENING
Microscopy has historically been inherently a descriptive endeavor and in fact it is frequently described as an art as well as a science. It is also becoming increasingly recognized that image-based scoring needs to be standardized for numerous medical applications. For example, for medical diagnoses, interpretation of medical images has been used since the 1950s to distinguish disorders such as cervical dysplasias and karyotyping [1]. Cameras used in microscopes during this era were able to capture an image, reduce the image data to a grid that was printed on a dot-matrix printer and integrated regional intensities to interpret shapes and features. In essence, these principles have not changed in 50 years, but the sophistication and throughput with which it is done has increased with advances in microscope and camera design and computational power. In the early 1990s, these advances were realized as automated acquisition and analysis of biological assays became more common.
Advances in automated microscopy, namely the automated movement of slides on the stage, focusing, changing fluorophore filters, and setting proper image exposure times, were also essential to standardizing and improving biomedical imaging. Automated microscopy was necessary to reduce the amount of time required of laboratory personnel to produce these images, which was a bottleneck for these studies, especially medical diagnoses. A team of scientists from Boston and Cambridge, Massachusetts described an automated microscope in 1976 that directly anticipated its use in subcellular microscopy and image analysis [2]. The microscope, and a processed image of a promyelocyte captured using the instrument, are shown in Figure 1.1.
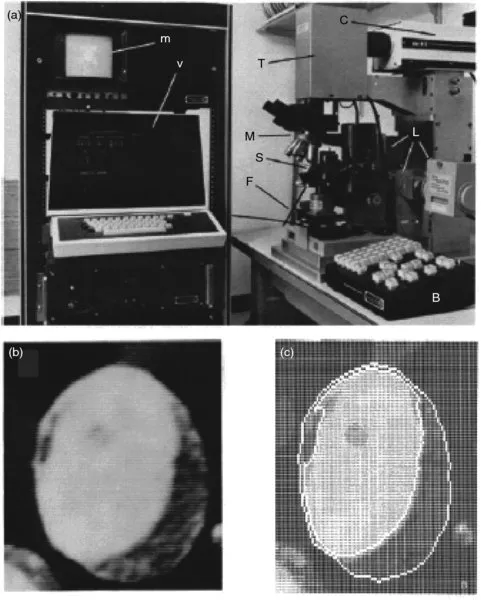
Figure 1.1 An early automated microscope used in biomedical research. (a) An example of an automated fluorescence microscope. Letters inside the figure are from the original source. The system is outfitted with controlled stage and filter movements (S and F), a push-button console for manual movements (B), a television camera and monitor (T and m) and a video terminal for digitizing video images (v). (b) A video image of a promyelocyte and (c) image analysis of (b), showing, an outline of the nucleus and cell borders, which can be used in automated cell type recognition. Reproduced with permission from [2]. Copyright 1974 John Wiley & Sons.
Until the mid-1990s, automated microscopy was applied in basic research to address areas of high technical difficulty, where rigorous measurements of subtle cellular events (such as textural changes) were needed, events that took place over long time periods or were rare (which made it challenging to acquire sufficient numbers of images of each event). In medicine, automated imaging was used to standardize the interpretation of assay results, such as for the diagnosis of disease from histological samples (where it was notoriously difficult to achieve concordance among clinical pathologists). Adapting quantitative imaging assays into a screening context was first described by Lansing Taylor and colleagues [3], who commercialized an automated microscope capable of screening samples in multiwell plates (a format that had emerged as an industry standard during this time period). The term âhigh contentâ was coined to contrast the low throughput screening in these imaging assays with the increasing scale of high throughput primary drug discovery screens. Many groups have since demonstrated the usefulness of automated microscopy in drug discovery [4, 5] and basic research [6, 7]. During this phase (the early 2000s), data acquisition, image analysis, and data management still imposed limits on image-based screening, but it did find an important place in the pharmaceutical industry, where expensive, labor-intensive assays critical for late-stage drug development were a bottleneck. One example is the micronucleus assay, an assay that measures the teratogenicity of novel therapeutics through counting the number of micronuclei (small nonnuclear chromosomal fragments that result from dysregulation of mitosis). An increase in the number of cells that contain micronuclei is indicative of genotoxicity, so this assay is frequently part of a screening program to make a go/no go decision on clinical development [8]. The assay requires finding binucleate cells and checking for a nearby micronucleus. For each compound assayed, a single technician might spend many hours in front of a microscope searching and counting nuclei. Automation of image capture and analysis not only reduced the work burden of researchers, but it also made the analysis itself more robust [9]. Similar applications were found in the field of cell biology, where automated microscopy was utilized to collect and analyze large data sets [10, 11].
Following from these early implementations, high content screening (HCS) has been widely adopted across many fields as the technology has improved and more instruments are available commercially. The speed at which images can be analyzed is limited by computer power, as more advanced computer technology has been developed, the scale at which samples can be analyzed has improved. Faster computers also mean that more measurements per cell can be made; shapes of cells and subcellular structures can be analyzed as well as probe intensities within regions of interest. This has led to the quantification of subtle morphological changes as assay endpoints. A widely used application of this approach has been receptor internalization assays, such as the Transfluor⢠assay to measure the activation of GPCRs through changes in the pattern of receptor staining, from even staining over the surface of the cells to dense puncta following internalization of the activated receptors through vesicle formation [12]. Concomitant with the increase in the sophistication of the assays themselves, improvements in the mechanical process of screening samples has also fed the growth of HCS. Gross-level changes, such as integrating plate handling robotics and fine-level changes, such as improvements in sample detection and autofocusing, have improved the scale of HCS to the point where image-based readouts are possible for true high throughput screens (screens of greater than 100,000 compounds) [5].
HCS has a strong presence in basic biological studies as well. The most widely recognized applications are similar to screening for drug candidates, including siRNA screening to identify genes that control a biological process, and chemical genetics, the identification of small molecules that perturb a specific cellular protein or process. While operationally similar to drug screening, they seek to explain and study biological questions rather than lead to therapeutics explicitly. Additional uses of HCS in basic science include the study of model organisms. Finally, the use of multiparametric single cell measurements has extended our understanding of pathway signaling in novel ways [11].
1.2 SIX SKILL SETS ESSENTIAL FOR RUNNING HCS EXPERIMENTS
At this point we want to touch on the fundamental skill sets required to successfully set up and use an HCS system to address a biological problem, and how responsibilities might be divided up in different settings. The six major skill sets required to develop and run an HCS project are shown in Figure 1.2. Each area is distinct enough as to be a full-fledged area of expertise (hence introducing these areas as âskill setsâ), but typically a person is competent in more than one area. It is rare that all roles can been successfully filled by one person. Therefore, the ability to develop a collaborative team is essential to HCS. It is also very important to understand that these roles vary between groups, and this can cause problems when people move between groups or as groups change in size. The skill sets are the following.

Figure 1.2 The basic skill sets essential for establishing and running HCS experiments. Skills noted in the figure are discussed in detail in the text.
1.2.1 Biology
The biologist develops the question that needs to be answered experimentally. In academia, the biologist is typically a cell biologist and oftentimes is also capable of collecting images by HCS as well. In industrial circles (pharma and biotech), a therapeutic team may be led by a biochemist or in vivo pharmacologist, who may have little training in fluorescence microscopy. The key area of expertise here is an appreciation of these problems and an ability to formulate strategies (experimental systems and assays) to address them. There is also a significant understanding of how cellular models in the laboratory relate to the biology in vivo. In addition to understanding the fundamental biological question, understanding how to establish a cellular model that incorporates relevant aspects of the biological environment is important.
1.2.2 Microscopy
Although many of the HCS systems are sold as turnkey âblack-boxes,â it is important to have a good understanding of fundamental microscopy components (staining techniques, reagents, and optics) as each has a significant impact on the quality of data generated by the instruments. For example, the choice of illumination system and filter sets determine which fluorescence wavelengths (fluorophores) you can use to stain specific cellular compartments. Other microscope objective characteristics (numerical aperture, magnification, and working distance) also impact both the types of samples one can image as well as the spatial resolution of the resulting images. More information on these topics are covered in Chapters 3 and 7. If the biological question is posed by someone who is not a trained microscopist, then it is important to discuss technical aspects with someone who has such training, which is why these skills are frequently part of the Platform Manager responsibilities (see below), particularly when the HCS instrument is used in a core facility.
1.2.3 HCS Instrumentation (Platform Manager)
The platform manager focuses on the hardware and software needed to keep an HCS facility running smoothly. Much of the information needed to run a particular HCS instrument is obtained from the instrument vendor, including instrument operation and, in some cases, strategies for data analysis; the Platform Manager will be the one who interacts with the vendors directly, particularly for handling challenging problems with the instrumentation or for scheduling updates to the instrument. Although the image acquisition configuration is often simplified by the user interface software, a solid understanding of imaging hardware (laser autofocus, CCD camera exposure time, PMT amplifier gain) is needed for optimal use of the instrument. In addition, it is important to know how to integrate the HCS instrument wi...
Table of contents
- Cover
- Titlepage
- Copyright
- PREFACE
- CONTRIBUTORS
- 1 Introduction
- SECTION I FIRST PRINCIPLES
- SECTION II GETTING STARTED
- SECTION III ANALYZING DATA
- SECTION IV ADVANCED WORK
- SECTION V HIGH CONTENT ANALYTICS
- Appendix A Websites and Additional Information on Instruments, Reagents, and Instruction
- Appendix B A Few Words About One Letter: Using R To Quickly Analyze Hcs Data
- Appendix C Hypothesis Testing for High Content Data: A Refresher
- GLOSSARY
- TUTORIAL
- INDEX
- END USER LICENSE AGREEMENT