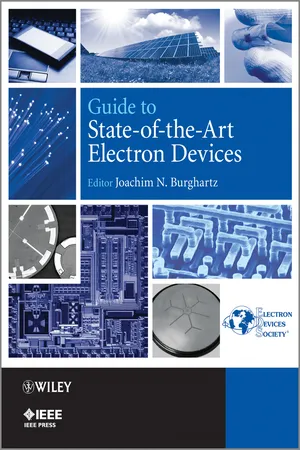
This is a test
- English
- ePUB (mobile friendly)
- Available on iOS & Android
eBook - ePub
Guide to State-of-the-Art Electron Devices
Book details
Book preview
Table of contents
Citations
About This Book
Winner, 2013 PROSE Award, Engineering and Technology
Concise, high quality and comparative overview of state-of-the-art electron device development, manufacturing technologies and applications
Guide to State-of-the-Art Electron Devices marks the 60th anniversary of the IRE electron devices committee and the 35th anniversary of the IEEE Electron Devices Society, as such it defines the state-of-the-art of electron devices, as well as future directions across the entire field.
- Spans full range of electron device types such as photovoltaic devices, semiconductor manufacturing and VLSI technology and circuits, covered by IEEE Electron and Devices Society
- Contributed by internationally respected members of the electron devices community
- A timely desk reference with fully-integrated colour and a unique lay-out with sidebars to highlight the key terms
- Discusses the historical developments and speculates on future trends to give a more rounded picture of the topics covered
A valuable resource R&D managers; engineers in the semiconductor industry; applied scientists; circuit designers; Masters students in power electronics; and members of the IEEE Electron Device Society.
Frequently asked questions
At the moment all of our mobile-responsive ePub books are available to download via the app. Most of our PDFs are also available to download and we're working on making the final remaining ones downloadable now. Learn more here.
Both plans give you full access to the library and all of Perlegoâs features. The only differences are the price and subscription period: With the annual plan youâll save around 30% compared to 12 months on the monthly plan.
We are an online textbook subscription service, where you can get access to an entire online library for less than the price of a single book per month. With over 1 million books across 1000+ topics, weâve got you covered! Learn more here.
Look out for the read-aloud symbol on your next book to see if you can listen to it. The read-aloud tool reads text aloud for you, highlighting the text as it is being read. You can pause it, speed it up and slow it down. Learn more here.
Yes, you can access Guide to State-of-the-Art Electron Devices by Joachim N. Burghartz in PDF and/or ePUB format, as well as other popular books in Technology & Engineering & Electrical Engineering & Telecommunications. We have over one million books available in our catalogue for you to explore.
Information
Part I
Basic Electron Devices
Click here to view the historic timeline
Chapter 1
Bipolar Transistors
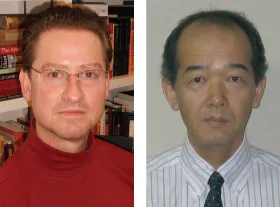
1.1 Motivation
In terms of its influence on the development of modern technology and hence, global civilization, the invention of the point contact transistor on December 23, 1947 at Bell Labs in New Jersey by Bardeen and Brattain was by any reckoning a watershed moment in human history [1]. The device we know today as a bipolar junction transistor was demonstrated four years later in 1951 by Shockley and co-workers [2] setting the stage for the transistor revolution. Our world has changed profoundly as a result [3].
Interestingly, there are actually seven major families of semiconductor devices (only one of which includes transistors!), 74 basic classes of devices within those seven families, and another 130 derivative types of devices from those 74 basic classes (Figure 1.1) [4]. Here we focus only on three basic devices: (1) the pn homojunction junction diode (or pn junction or diode), (2) the homojunction bipolar junction transistor (or BJT), and (3) the special variant of the BJT called the silicon-germanium heterojunction bipolar transistor (or SiGe HBT). As we will see, diodes are useful in their own right, but also are the functional building block of all transistors.
Figure 1.1 The transistor âfood chainâ showing all major families of semiconductor devices.
Reproduced with permission from Cressler, J. D.; Silicon Earth: Introduction to the Microelectronics and Nanotechnology Revolution; 2009, Cambridge University Press
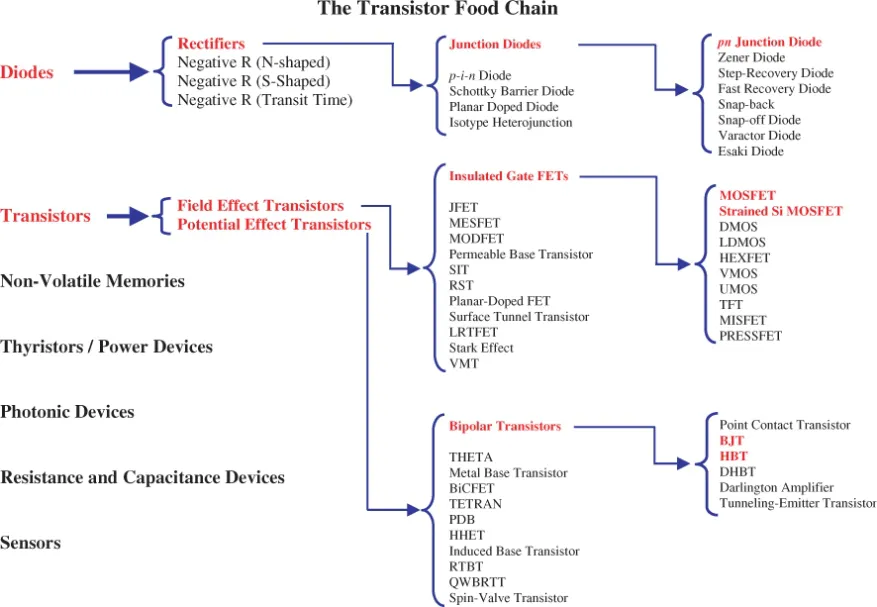
Surprisingly, all semiconductor devices can be built from a remarkably small set of materials building blocks (Figure 1.2), including [4]:
- the metalâsemiconductor interface (e.g., Pt/Si; a âSchottky barrierâ)
- the doping transition (e.g., a Si p-type to n-type doping transition; the pn junction)
- the heterojunction (e.g., n-AlGaAs/p-GaAs)
- the semiconductor/insulator interface (e.g., Si/SiO2)
- the insulator/metal interface (e.g., SiO2/Al).
Click here to view the historic timeline
Figure 1.2 The essential building blocks of all semiconductor devices.
Reproduced with permission from Cressler, J. D.; Silicon Earth: Introduction to the Microelectronics and Nanotechnology Revolution; 2009, Cambridge University Press
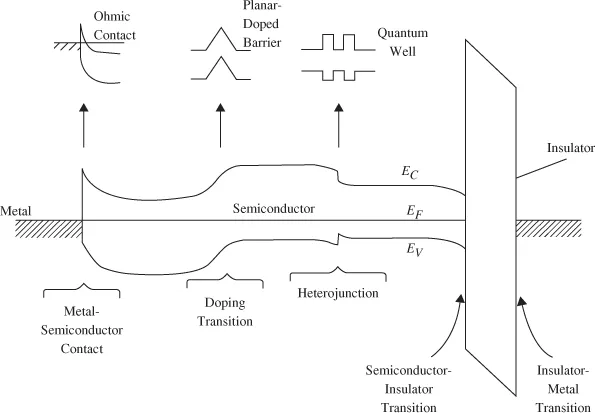
Naming of the Transistor
The name âtransistorâ was actually coined by J.R. Pierce of Bell Labs, following an office betting pool which he won. He started with a literal description of what the device actually does electronically, a âtransresistance amplifier,â which he first shortened to âtrans-resistor,â and then finally âtransistorâ [3].
Why do we actually need transistors in the first place? Basically, because nature attenuates all electrical signals. By this we mean that the magnitude of all electrical signals (think â1sâ and â0sâ inside a computer, or an EM radio signal from a cell phone) necessarily decreases as it moves from point A to point B, something we call âlossâ. When we present an (attenuated) input signal to the transistor, the transistor is capable of creating an output signal of larger magnitude (i.e., âgainâ), and hence the transistor serves as a âgain blockâ to âregenerateâ (recover) the attenuated signal in question, an essential concept for electronics. In the electronics world, when the transistor is used as a source of signal gain, we refer to it as an âamplifier.â Amplifiers are ubiquitous to all electronic systems.
Click here to view the historic timeline
Not only can the transistor serve as a wonderful nanoscale sized amplifier, but importantly it can also be used as a tiny âregenerative switchâ; meaning, an on/off switch that does NOT have loss associated with it. Why is this so important? Well, imagine that the computational path through a microprocessor requires 1 000 000 binary switches (think light switch on the wall â on/off, on/off) to implement the complex digital binary logic of a given computation. If each of those switches even contributes a tiny amount of loss (which it inevitably will), multiplying that tiny loss by 1 000 000 adds up to unacceptably large system loss. That is, if we push a logical â1â or â0â in, it rapidly will get so small during the computation that it gets lost in the background noise. If, however, we implement our binary switches with gain-enabled transistors, then each switch is effectively regenerative, and we can now propagate the signals through the millions of requisite logic gates without excessive loss, maintaining their magnitude above the background noise level.
In short, the transistor can serve in one of two fundamental capacities: (1) an amplifier or (2) a regenerative switch. Amplifiers and regenerative switches work well only because the transistor has the ability to produce gain. So a logical question becomes, where does transistor gain come from? To answer this, first we need to understand pn junctions.
1.2 The pn Junction and its Electronic Applications
Virtually all semiconductor devices (both electronic and photonic) rely on pn junctions (a.k.a., âdiodesâ, a name which harkens back to a vacuum tube legacy) for their functionality. The simplest embodiment of a pn junction is the pn âhomojunctionâ, meaning that within a single piece of semiconductor (e.g., silicon â Si) we have a transition between p-type doping and n-type doping (e.g., p-Si/n-Si). The opposite would be a pn heterojunction, in which the p-type doping is within one type of semiconductor (e.g., p-GaAs), and the n-type doping is within another type of semiconductor (e.g., n-AlGaAs).
Click here to view the historic timeline
As shown in Figure 1.3, to build a pn junction we might, for instance, ion implant and then diffuse n-type doping into a p-type wafer. The important thing is the resultant âdoping profileâ as one moves through the junction (ND(x) â NA(x), which is just the net doping concentration). At some point in the doping transition, ND = NA, and we thus have a transition between net n-type and net p-type doping. This point is called the âmetallurgical junctionâ (x0 in Figure 1.3) and all of the important electrical action of the junction is centered here. To make the physics easier, two simplifications are typically made: (1) Let us assume a âstep junctionâ approximation to the real pn junction doping profile, which is just what it says, an abrupt change (a step) in doping occurring at the metallurgical junction (Figure 1.3). (2) Let us assume that all of the dopant impurities are ionized (one donor atom equals one electron, etc., an excellent approximation for common dopants in silicon at 300 K).
Figure 1.3 Cartoons of a pn junction, showing doping transition from n-type to p-type.
Reproduced with permission from Cressler, J. D.; Silicon Earth: Introduction to the Microelectronics and Nanotechnology Revolution; 2009, Cambridge University Press
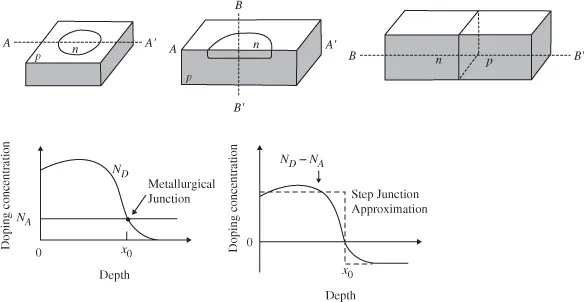
So, how does a pn junction actually work? The operation of ALL semiconductor devices is best understood at an intuitive level by considering the energy band diagram, which plots electron and hole energy as a function of position as we move physically through a device. An n-type semiconductor is electron rich (i.e., majority carriers), and hole poor (i.e., minority carriers). Conversely, a p-type semiconductor is hole-rich and electron-poor. If we imagine bringing an n-type and p-type semiconductor into âintimate electrical contactâ where they can freely exchange electrons and/or holes from n to p and p to n, the final equilibrium band diagram shown in Figure 1.4 will result. Note, that under equilibrium conditions, there is no NET current flow across the junction.
Figure 1.4 Energy band diagram of a pn junction at equilibrium.
Reproduced with permission from Cressler, J. D.; Silicon Earth: Introduction to the Microelectronics and Nanotechnology Revolution; 2009, Cambridge University Press
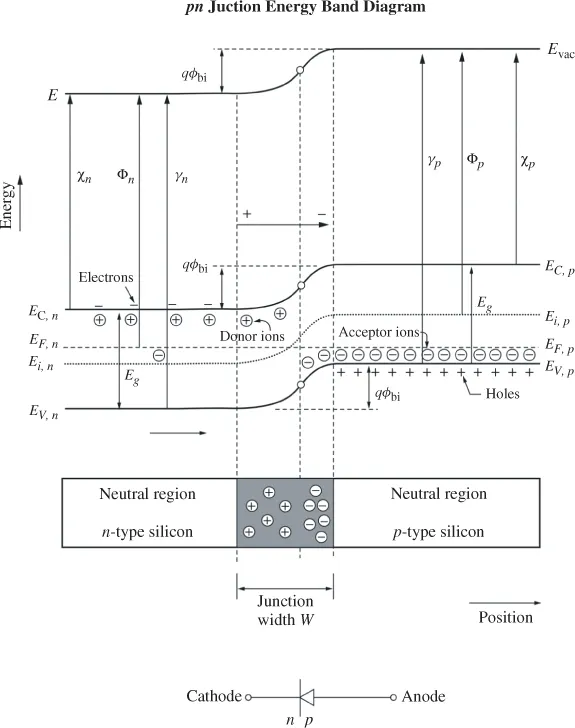
Click here to view the historic timeline
We might logically wonder what actually happened inside the junction to establish this equilibrium condition. When brought into contact, the n-type side of the junction is electron rich, while the p-type side is electron poor. That is, there is a large driving force for electrons to diffuse from the n region to the p region. Recall, that there are in fact two ways to move charge in a semiconductor: (1) drift, whose driving force is the electric field (voltage/length), and (2) diffusion, whose driving force is the carrier density gradient (change in carrier density per unit distance). The latter process is what is operative here. Once in electrical contact an electron moves from the n-side to the p-side, leaving behind a positively charged donor impurity (ND+). Note, that far away from the junction, for each charged donor impurity there is a matching donated electron, hence the semiconductor is charge neutral. Once the electron leaves the n-side, however, there is no balancing charge, and a region of âspace chargeâ results. The same thing happens on the p-side. Hole moves from p to n, leaving behind an uncompe...
Table of contents
- Cover
- Title Page
- Copyright
- Foreword
- Preface
- Contributors
- Acknowledgments
- Introduction: Historic Timeline
- Part I: Basic Electron Devices
- Part II: Aspects of Device and IC Manufacturing
- Part III: Applications Based on Electron Devices
- Index